THOUGH the existence of
ironstone in the Scotch coal measures was known many years previously, no
attempt was made to turn it to account until the year 1760, when the Carron
Ironworks were established. Only one kind of ironstone was then used—namely,
the argillaceous or "clayband;" for the more valuable carbonaceous or "blackband"
was not discovered till the beginning of the present century. These two
varieties are known as the coal measure iron-stones, and are found in all
the great coal fields of Britain except those of Northumberland, Durham, and
Lancashire. Though there are nineteen kinds of iron ore known to the
mineralogist, it has been calculated that nine-tenths of the iron produced
is derived from the clayband and blackband ironstones, the relative value of
which is thus stated in a paper read before the Scottish Society of Arts by
Mr Ralph Moore, Government inspector of Mines—"Clay ironstones contain from
thirty to fifty per cent. of metallic iron. Before being melted, they are
mixed with coal, and calcined in kilns or large heaps, to drive off the
carbonic acid gas, sulphur, and other impurities. This description of
ironstone is found in seams or bands, and in nodules, throughout the whole
of the measures, but is most plentiful in the lower part of the section.
Blackband ironstone is a carbonate of iron, laminated with coal, generally
in sufficient quantity for calcination without further admixture of coal;
and leaves, when calcined, a metallic coke containing from fifty to seventy
per cent. of metallic iron. This description of ironstone is found in seams
or bands in well-defined positions in the measures, but these are neither
persistent in position nor equable in quality. Sometimes the seam is wanting
altogether, or so thin as to be unworkable; at other times, the coaly
element so predominates that its metallic value is of small amount, while
not unfrequently it contains nothing but coal. A good blackband ironstone
contains from two to eight per cent. of coal. When it contains more than
twenty per cent of coal, it is of little value unless mixed with clayband,
which uses up the excess of coal. It is more easily melted than clayband,
and requires less coal; and the weekly produce of a furnace from blackband
is fifty per cent. greater than from clayband."
Deposits of hematite, or red
iron ore, have been discovered recently in Haddingtonshire, Dumfriesshire,
and Kirkcudbright, and operations for utilising the ore are in progress.
From the establishment of the
Carron Ironworks in 1760 till 1788, the quantity of iron produced in
Scotland did not exceed 1500 tons per annum; but during the succeeding eight
years a number of new furnaces were erected in the counties of Lanark, Fife,
and Ayr. In 1796 the number of furnaces was seventeen, and the quantity of
iron made in that year was 18,640 tons. Thirty-three years afterwards the
production had reached 29,000 tons; and from that figure the invention of
the hot-blast process raised it to 75,000 tons in 1836. By that time the
construction of railways had begun to open a new market for the
iron-merchant; and to supply the demand, many new iron-stone pits were
opened, and furnaces erected. In the ten years from 1835 to 1845, the
production of iron increased about 700 per cent.— the quantity made in the
latter year being 475,000 tons. Ten years later, 825,000 tons were made; and
another decade gave an addition of 339,000—the quantity manufactured in 1865
being 1,164,000 tons. Over speculation, and the consequent financial crisis
in 1866, operated most prejudicially on the iron trade, and the production
for that year fell to 994,000 tons. Matters gave promise of taking a turn
for the better in 1867; but the promise was realised only to a limited
extent, the revelations made in connection with several of the principal
railway companies, and other causes, having had an unfavourable effect on
the trade. The total quantity made in 1867 was 1,031,000 tons—an increase of
37,000 as compared with the preceding year, but 133,000 tons short of the
production of 1865. The quantity shipped in each of the past ten years has
not varied much. In 1867 it was 593,277 tons, of which 338,364 tons were for
foreign ports, and the remainder went coastwise. Of the quantity shipped to
foreign ports, France took 60,500 tons; Germany and Holland 99,600 tons;
Belgium, Denmark, Sweden, and Norway, 20,100; Russia, 9600; Spain and
Portugal, 5100; Italy, 14,200; United States, 117,300; British America,
43,000; East Indies, China, Australia, and South America, 14,000. The
largest quantity ever consumed in Scotland in one year was 532,000 tons, in
1865. In 1867, the quantity taken was 420,262 tons. The make of malleable
iron in 1867 was 142,800 tons, being a reduction of 11,400 tons as compared
with 1866.
The rapid development of the
iron trade has not been peculiar to Scotland. England, Wales, and a number
of Continental countries have had a similar experience, arising from the
same causes—namely, the formation of railways, the substitution of iron for
wood in the construction of ships, and its increased application, in the
form of machinery, to the industrial arts. The number of blast furnaces in
Scotland is 164, of which 108 were in blast during 1867. Each furnace
produced on the average about 9546 tons. One furnace gives employment,
directly and indirectly, to fully two hundred men and boys, so that the
number of persons engaged in the production of pig-iron dining the year
could not be less than 22,000, with wages ranging from 2s. to 6s. a-day. It
will thus be seen that the " damping out," or stoppage of a furnace is a
serious matter for the working population in the iron districts. Were the
entire number of furnaces in blast, employment would be given to upwards of
33,000 men and boys, while the annual production would exceed 1,500,000
tons. The number of ironstone miners in Scotland is about 13,000, and the
largest quantity of ore put out in a year was 2,500,000 tons in 1857.
The occupation of the
ironstone miner differs little from that of the coal-miner, and the two
occupy nearly the same position as regards wages, &c. The ironstone seams
are generally only from six to eighteen inches in thickness, so that in
taking out the ore a considerable quantity of rock has to be excavated. As
the miner advances, he builds up behind him as much as possible of the stone
and rubbish, and sends out the ironstone and surplus material in small
waggons or "hutches."
The price of pig-iron has
been subject to considerable fluctuations. In 1854, the mean average price
of a ton of Scotch pig-iron was 79s. 7d.; it was 54s. 4d. in 1858; and 49s.
3d. in 1861. In 1866 the market was much disturbed by the operations of
certain bold speculators, who forced the top price up to 82s. 6d.—an
increase of 21s. on the price at the close of the preceding year. Then came
financial disasters; and the brief space of four weeks witnessed a fall of
31s. 6d. a ton. After rising and falling several times subsequently,
the price at the close of 1866 was 54s. 6d. In order to enable them to
overcome the effects of the crisis brought about by the speculators alluded
to, the ironmasters resolved to reduce the production, and forty furnaces
were "blown out." Nearly eight thousand men and boys were thus thrown idle,
while at the same time the wages of the men retained were considerably
reduced. In the course of 1867 a number of the furnaces which had been
stopped were set on, and about one-third of the men who had been thrown idle
were restored to work. The average price of pig-iron during 1867 was 53s.
6d. per ton. The lowest figure reached in the course of the year was 51s.
3d., in the month of March, and the highest 55s. 6d., in October.
From the convenient situation
and facilities for transport enjoyed by Scotch ironmasters, coupled with the
cheapness of labour, it might be thought that no English or other producers
of the metal could undersell them; but it is, nevertheless, a fact that
something like 70,000 tom of pig-iron were in 1867 imported into Scotland,
from Middlesborough, in Yorkshire, where ironstone costs less than
one-fourth of its value in Scotland. The iron is, however, of a much lower
quality than the native Scotch, and is used for mixing with the latter for
the production of certain kinds of material. A small quantity of fine
pig-iron is brought from West Cumberland by some of the malleable iron
makers, who use it to mix with and improve the quality of the native iron.
It is expected that, when the Solway Junction Railway is opened, a large
quantity of Cumberland iron ore will be brought into Scotland. In 1868 the
price of malleable bars ranged from L.6, 15s. to L.7, 5s. a ton; plates,
L.8, 10s.; and rails, L.6 to L.7. Cast-iron pipes were quoted at from L.4,
15s. to L.6 per ton, and railway chairs at from L.3, 12s. 6d. to L.4.
The most valuable deposits of
ironstone are in Lanarkshire and Ayrshire, and in the former county
two-thirds of the pig-iron made in Scotland is produced. The blast furnaces
are chiefly concentrated in the vicinity of Coatbridge, Airdrie, and Wishaw,
all of which towns were rapidly raised to importance by the development of
the mineral treasures which lay beneath and around them. Coatbridge stands
within a crescent of blast furnaces, and in the town are a large number of
rolling mills, forges, and tube works, the hundred chimneys of which form
quite a forest of brickwork capped with fire.
Though Coatbridge is a most
interesting seat of industry, it is anything but beautiful, Dense clouds of
smoke roll over it incessantly, and impart to all the buildings a peculiarly
dingy aspect. A coat of black dust overlies everything, and in a few hours
the visitor finds his complexion considerably deteriorated by the flakes of
soot which fill the air, and settle on his face. To appreciate Coatbridge,
it must be visited at night, when it presents a most extraordinary and—when
seen for the first time—startling spectacle. From the steeple of the parish
church, which stands on a considerable eminence, the flames of no fewer than
fifty blast furnaces may be seen. In the daytime these flames are pale and
unimpressive; but when night comes on, they appear to burn more fiercely,
and gradually there is developed in the sky a lurid glow similar to that
which hangs over a city when a great conflagration is in progress. For
half-a-mile round each group of furnaces, the country is as well illumined
as during full moon, and the good folks of Coatbridge have their streets
lighted without tax or trouble. There is something grand in even a distant
view of the furnaces; but the effect is much enhanced when they are
approached to within a hundred yards or so. The flames then have a
positively fascinating effect. No production of the pyrotechnist can match
their wild gyrations. Their form is ever changing, and the variety of their
movements is endless. Now they shoot far upward, and breaking short off,
expire among the smoke; again spreading outward, they curl over the lips of
the furnace, and dart through the doorways, as if determined to annihilate
the bounds within which they are confined; then they sink low into the
crater, and come forth with renewed strength in the shape of great tongues
of fire, which sway backward and forward, as if seeking with a fierce
eagerness something to devour.
The most extensive
ironmasters in Scotland are Messrs Baird and Co., who own forty-two blast
furnaces, employ nine thousand men and boys, and produce about three hundred
thousand tons of pig-iron per annum, or one-fourth of the entire quantity
made north of the Tweed. Twenty-six of their furnaces are situated in
various parts of Ayrshire, and the remaining sixteen are concentrated at
Gartsherrie, in the neighbourhood of Coatbridge. Gartsherrie Ironworks are
the largest in Scotland, and it is stated there is only one establishment in
Britain which has a greater number of furnaces. The quantity of pig-iron
made is one hundred thousand tons per annum, and the number of men and boys
connected with the works is three thousand two hundred. More than a thousand
tons of coal are consumed every twenty-four hours; and, as showing how well
chosen is the site of the works, it may be mentioned that
nineteen-twentieths of the coal required is obtained within a distance of
half-a-mile from the furnaces. One coal-pit is situated close to the
furnaces, and has been in operation since the works were established, forty
years ago.
The coal from this pit is
conveyed to the furnaces by means of a self-acting incline. Most of the
ironstone was at one time obtained from pits in the nighbourhood, but now it
has to be brought from a distance of from two to twenty miles; and a
complete system of railways connects the pits with the works. The total
length of the railways is about fifty miles, and the traffic is carried on
by means of six locomotives and an immense number of trucks. The
establishment is also connected with the great railway systems of the
country, and possesses additional facilities for transport in a branch of
the Monklands Canal, which has been carried through the centre of the works.
For the canal traffic, there is a fleet of eighteen barges, of about sixty
tons each; and eight of these are screw steamers. A great proportion of the
manufactured iron is sent out by the canal.
As the Gartsherrie Ironworks
have a wide-spread reputation for producing iron of a superior quality, and
are among the best organised manufactories in the country, a description of
them may be interesting.
The furnaces, sixteen in
number, stand in two rows, one on each side of the canal, and about forty
yards distant from it. A constant supply of coal and ironstone can be
reckoned upon, and therefore only a small stock is kept at the works. The
mineral trains are worked with unfailing regularity, and their cargoes are
deposited conveniently for immediate use. There is thus no superfluous
shovel¬ling about of the materials, nor is any expense incurred by piling
them into heaps. The proportions of ironstone, coal, and limestone, laid
down are exactly what are required in the process of smelting. Manual labour
has, by a variety of ingenious appliances, been reduced to a minimum, and
the amount of waste is infinitesimal. Everything is done according to a
well-defined system, and nothing connected with the works is considered to
be too insignificant to merit attention. No heaps of rubbish are allowed to
accumulate, no scraps of iron or cinder lie about, and every nook and cranny
about the vast place is as tidily kept as it can possibly be. The workmen
are liberally treated, but they must do their work carefully and well.
Negligence and irregularity are unfailingly punished, while merit is as
certainly rewarded. All the men employed about the furnaces, even the
firemen and engineers of the blast engines, are paid accord¬ing to the
quantity and quality of iron produced. This arrangement is found to work
admirably, as each man knows that, by attending to his work, he is not only
putting money into the pockets of his fellow-labourers, but also improving
his own earnings.
Before the ironstone is ready
for smelting it has to be calcined, which operation is performed at the
pits. The object of calcining is to separate carbonic acid, water, sulphur,
and other deleterious substances, which are volatile at a red heat; and it
is performed in this way :—A layer of rough coal is first laid down, and on
that the ore, mixed with a certain quantity of small coal, is piled. The
blackband ironstone, as it contains a large proportion of carbon, requires
less coal to calcine it than the clayband. When the heap is completed, fire
is applied to the windward side, and combustion goes on gradually until the
desired effect is produced. When the ore cools, it is ready for the furnace;
but when the heat has been too intense, the ore is found to have run into
large masses, the breaking up of which takes a considerable amount of
labour.
Having been built at
different periods, the Gartsherrie furnaces are of various patterns. The
general shape is cylindrical, the diameter twenty-two feet, and the height
sixty feet. The Nelson Monument, on Calton Hill, Edinburgh, would, were it
less lofty, bear a close resemblance to one of the most recently erected
furnaces. The furnace is fed from the top, and, in order to protect the "
fillers," the mouth of it is surrounded by a light wall of brick, pierced
with convenient openings. This brick wall is so much thinner than the main
wall of the furnace on which it stands, that a gallery or foot¬way several
feet in width is left clear all round. Externally, there are four arched
recesses in the base of the furnace, three of which are occupied by the "tuyeres,"
or pipes conveying the "blast;" while the fourth contains a doorway by which
the "slag" is drawn off, and also the opening through which the molten iron
is discharged. The interior of the furnace consists of a circular cavity,
seven and a half feet in diameter at the lower part or hearth. At a height
of five or six feet from the bottom of the hearth, the cavity begins to
increase in diameter, until, at half the height of the furnace, it measures
eighteen feet across. It is then gradually contracted, and at the top the
diameter is eleven feet. The materials with which the furnace is fed are
roasted ore, coal, and limestone. The proportions of these vary according to
their quality. In some cases, a small quantity of red-iron ore or hematite
is used along with the blackband ironstone, and then the proportions of what
are called a "charge" are these:—Coal, about 10 cwt.; roasted ore, 6 cwt.;
red ore, cwt.; and lime, 21 cwt. About sixty "charges" are thrown into the
furnace in the course of twelve hours, and at six o'clock in the morning and
at six at night the furnace is " tapped" and the iron ran off. The chemical
changes undergone by the materials introduced into the furnace are thus
described:—The iron ore consists of iron, oxygen, and sand, and the object
of the iron smelter is to separate the two latter substances from the
former. The coal introduced has two functions to fulfil—in part it is burned
so as to raise the contents of the furnace to such a high temperature that
they will be enabled to act on each other; and, at the same time, it carries
away the oxygen which was originally in combination with the iron in the
roasted ore. The lime plays the part of a flux, and combines with the sandy
matter to form a slag. During the whole operation, hot air is being
constantly forced in at the lower part of the furnace, so as to aid in the
necessary combustion. The roasted iron ore being thus deprived of its oxygen
by the coal, and of its sand by the lime, allows the other constituent—the
iron—to trickle down through the mass of red-hot cinders to the lower part
or hearth of the furnace.
In front of each furnace is a
level piece of ground covered with coarse sand, in which before the "
tapping" takes place a number of small furrows are formed. These communicate
with larger channels leading from the opening in the furnace; and when the
iron is let out, it runs along the main channels in a glowing, bubbling
stream, and distributes itself into all the hollows. The large channels are
called "sows," and the small ones "pigs;" hence the term "pig-iron." Two men
are employed to feed each furnace. One fills half a charge of coal into a
large iron barrow, and the other half a charge of the other materials into a
second barrow. The men and the barrows reach the staging communicating with
the mouth of the furnace by means of a hydraulic lift. The coal is thrown in
first, and the other materials immediately afterwards. The occupation of the
"fillers " appears to be a somewhat dangerous one, as the flames at times
shoot out upon, and almost surround them. Two men are employed at the hearth
scooping out the slag and cinders with a huge spoon suspended from a crane,
and from time to time stirring up the contents of the furnace. This is very
severe labour, and the faces of the men engaged in it have a half-roasted
appearance. The slag is poured into iron trucks, and, when it consolidates,
is wheeled away to be emptied on the waste heap—which, it may be mentioned,
contains as much material as would build a copy of the Great Pyramid. The
pig moulds are formed in the sand by boys, the operation being a very simple
one.
Up till about forty years ago
the air forced into blast-furnaces was cold, and the process of smelting was
slow, and also costly, in consequence of the great quantity of coal that was
required. In 1827, Mr J. B. Neilson, engineer of the Glasgow Gas-Works,
conceived the idea of heating the air before injecting it into the furnace;
and two years afterwards a most successful trial was given to the invention
at the Clyde Ironworks. With the cold blast coke had to be used, and 8 tons
11 cwt. of coal converted into coke was required to reduce one ton of iron.
It was found that when heated air was employed the coal might be used raw,
and that 2 tons 13f cwt. was sufficient to smelt a ton of iron, including 8
cwt. required for heating the air. This discovery gave an extraordinary
impetus to the iron trade, and the patentee and his partners are said to
have realised L.300,000 by the invention. At Gartsherrie there are three
immense engines for generating the blast—two for one range of furnaces, and
one for the other. The engines are on the beam principle, and their united "
duty" is equal to about 500 horse power. The steam cylinder of the largest
is five and a-half feet in diameter, and ten feet deep, and the air cylinder
is ten feet in diameter and depth. The air cylinders are simply gigantic
pumps, which force the air into receivers, whence it flows at an equal
pressure through the tubes of the heating oven, and into the furnace. By
passing through the oven the temperature of the air is raised to 800°. It
has been calculated that the quantity of air thrown into a blast-furnace in
full work exceeds in weight all the solid material used in smelting.
In the vicinity of
Gartsherrie there are about five hundred houses belonging to Messrs Baird &
Co., and occupied by their workmen. Nearly all the houses have two
apartments, and a few have a third room. A bit of garden ground is attached
to each house, and all are supplied with water and gas at a cheap rate. The
miners get as much coal as they require without payment—only they must dig
it out for themselves; and the other workmen are charged only 3s. 6d. for a
cartload. Liberal provision is made for the education of the children of the
workpeople. There are three schools in direct connection with the works,
each being divided into separate apartments for infants, boys, and girls.
The workmen seem to appreciate highly all that has been done for their
welfare, and few of them leave the place. They own one of the most
successful co-operative stores in the country. It is managed by a committee
of the workmen, but its prosperity is in a great measure owing to the
fostering care of the employers, who, however, have no interest in the
concern beyond seeing that it is properly conducted. There are seven hundred
members in the society, nearly all of whom are heads of families, and the
business done amounts to about L.1200 a-month. In addition to general
grocery goods, wines, spirits, butcher meat, and potatoes, are sold in the
store.
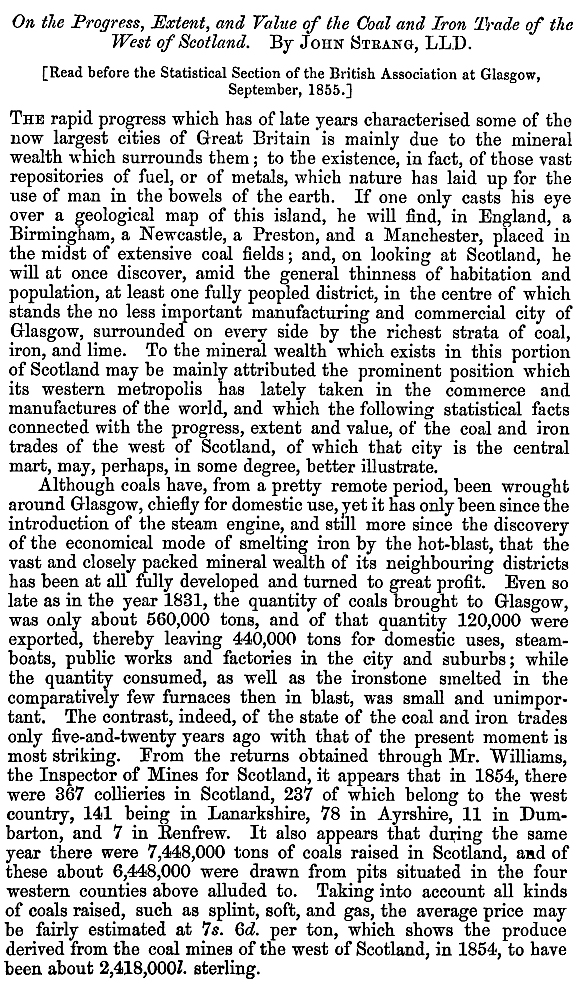
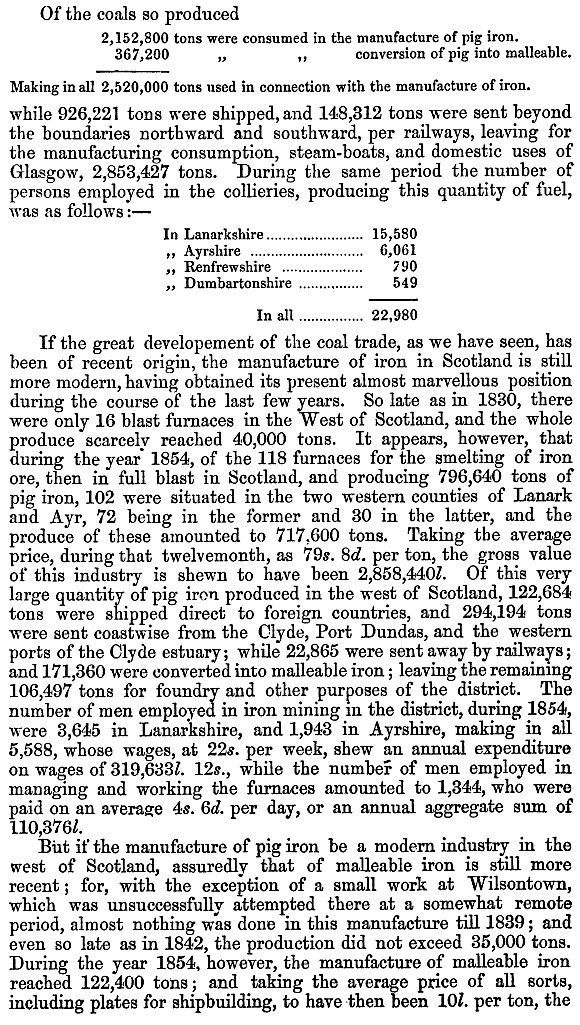
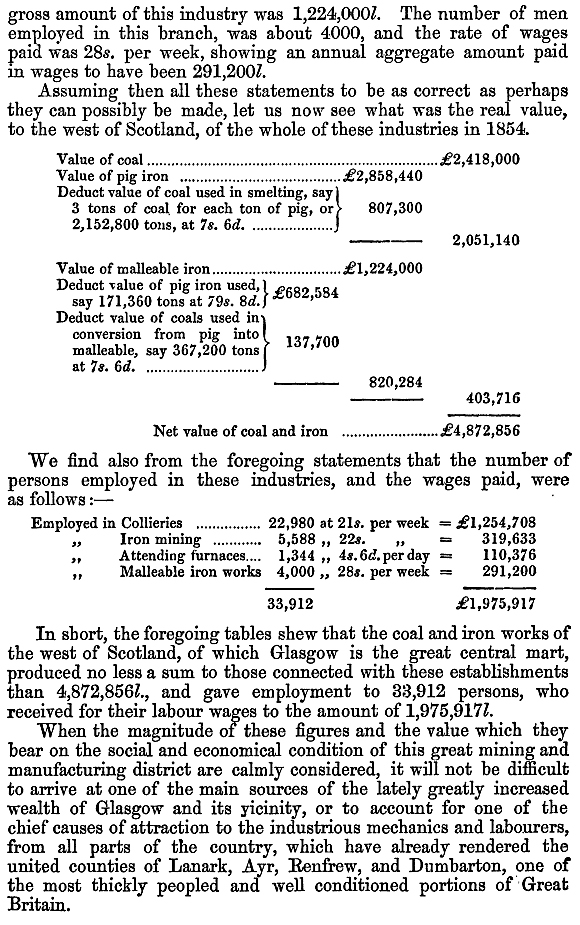
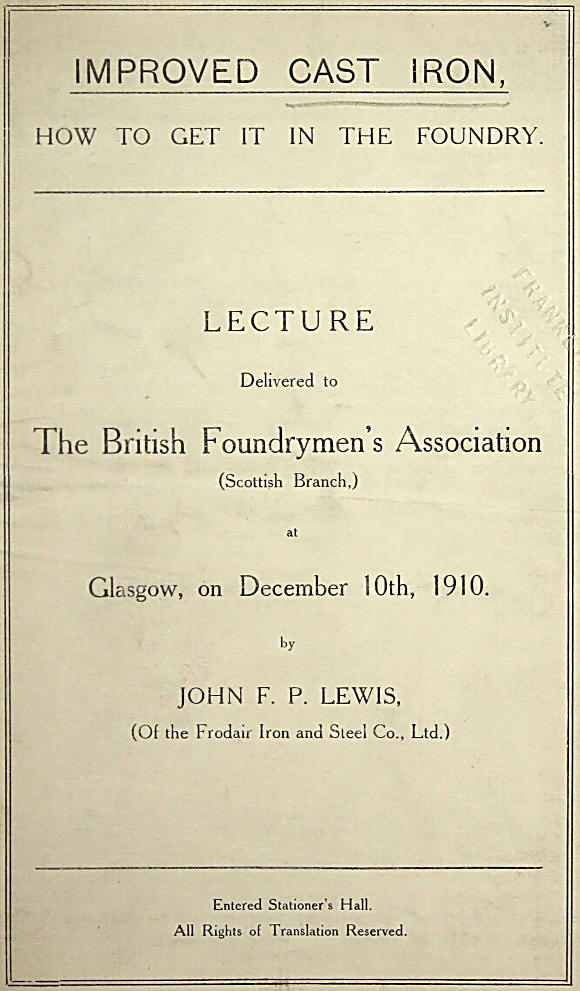
Of the many problems with which the ironfounder of to-day is
faced perhaps the most important, and certainly the most interesting, is
this: Can the quality of Cast Iron be improved to an extent sufficiently to
raise its value and extend its function in the eyes of the mechanical
engineer? 1 claim that the answer to this question is: Yes. 1 want to show
that this question has proper warrant for its introduction into the
deliberations of this Association; that touching the interests of the
ironfounding industry it is a useful and even a vital question.
During recent years Cast Iron has been gradually superseded
tor many important component parts of mechanical constructions by other
metals and alloys. The question is : Is this entirely the result of the
establishment of strength or physical quality specifications which under any
circumstances would have put Cast Iron out of court, or only partly the
result of that and partly the result of failures on the part of Cast Iron
owing to its being inferior in its own kind or defectively manufactured.
Now, in answering this, it is not possible in this lecture to take you over
the whole range of mechanical constructions and discuss where or where not
it would be possible to employ Cast Iron; it will be sufficient to cite one
or two concrete instances in which there are good practical reasons for
supposing that Cast Iron might have held against steel or wrought iron or
phosphor-bronze, or some other more costly metal or alloy, certain ground
which it has actually lost. 1 can, for instance, find hydraulic cylinders
made of steel which might have been constructed of Cast Iron without an
unwieldy section had the founder been able to guarantee a certain tensile
strength well within the known compass of Cast Iron. I can find working and
bearing parts of engines, such as slidebars, motion-blocks, eccentrics,
etc., made of steel or case-hardened wrought iron where Cast Iron of the
right hardness, tenacity and density would have been equally, if not more,
satisfactory. I can find steel, etc., being more and more specified for
superheated steam fittings, whilst careful experimenting shows that Cast
Iron of the best quality is just as suitable. I can find the more
homogeneous and expensive metals in use for parts of meters, freezing plant
and pneumatic engines, where iron is quite capable of meeting the
necessities as regards density and strength, and I can sometimes find highly
expensive alloys used for pots, retorts, kettles and other vessels subject
to strong acid or alkaline reactions, or to the penetrating or erosive
action of fumes, gases and vapours, where iron of the right chemical
composition and properly manufactured would be at any rate a much more
economical material. The improved Cast Iron suitable for work of this
description must be superior to ordinary commercial qualities in the
following respects : It must have a much higher specific weight; it must be
freer from those impurities, particularly free carbon, which interrupt the
crystalline continuity of its structure; it must be more regular in its
structure over large masses, that is to say it must, though it cannot
strictly be so, be much more nearly similar in physical character to the
metallic condition known as solid solution.
An ordinary commercial Cast Iron will have a tensile strength
of 8 or 9 tons per square inch, and a specially good iron will have a
tensile strength of 12 tons, and the average specific density of grey iron
castings will be about 7. It the ironlounder can turn out with certainty an
iron having a constant tensile strength of 15 or 16 tons and a constant
specific density of 7.25, he will hnd that he has a material capable of
competing with steel in many spheres where that metal now holds the field;
providing this iron possesses certain wearing qualities which will be
exhibited in its resistance to shock or abrasion, or to erosive actions, or
to variations of temperature, or to intermittent stresses, and this
combination of high tensile strength and high specific weight will almost
certainly mean that it does possess these qualities.
I am exhibiting here this evening a few specimens of Cast
Iron which have shewn under careful test the tensile strength and specific
weights mentioned. The official figures relating to these tests accompany
the samples, and the only further comment I need make on these samples is
that this material is quite easily machinable with good tools.
I want to show how such an iron can be turned out
commercially in the foundry, and first of all I must say that it is not a
question of getting these results once, but always. It is quite common in
the foundry to get an exceptional result occasionally, but a series of tests
nearly always shews a very great variation between the maximum and minimum
results, and outputs of iron which show wide ranges of quality are worse
than useless, and in my opinion are a particular source of danger to the
trade. It is these wide variations in quality which prejudice Cast Iron,
since the engineer with an unreliable material must base his calculations on
the lowest quality he has cognizance of in the material delivered to him.
It, therefore, has no influence on the Engineer if he is told a certain
iron will give up to 16 tons tensile as long as he knows that other
specimens in the same batch may only give 8 tons, or that one casting is
hard and tight as a drum if he finds that another is soft and porous, and
this irregularity in Cast Iron is at the root ol the whole question and
points the morals, since it means either that the founder’s raw material is
variable in quality or that his melting and manufacturing processes are
insufficiently understood or unscientifically controlled, or that both his
raw material and his processes are variable and defective.
Now, first of all, I w^ould like to discuss the material from
which our high grade iron can be produced, and afterwards the methods which
should be followed in producing it. I, therefore, must plunge now into the
much vexed question of pig iron and pig iron mixtures. I think we may
classify our choice of raw materials in 3 great headings :—(1) Consisting of
pig irons of first smelting and scrap iron (i.e., pig once cast); (2)
consisting of either part pig iron and part pig iron or pig iron and scrap
re-melted, or wholly of remelted pig iron or of re-melted pig and scrap; (3)
of any of the preceding along with steel or wrought iron in any shape or
form. Of these three I am going strongly to advocate the
first, i.e., mixtures of pig iron proper and scrap, and I will give reasons
for choosing this system and for rejecting the others. With reference to the
mixing of steel, wrought iron, etc., my chief objection to this is that it
throws a responsibility on the staff and plant in the foundry which the
foundry staff and plant are often unable to sustain. And here I wish it to
be specially understood that 1 am not addressing myself to specialists here
nor to those who are exceptionally skilful in foundry arts, nor to those
whose foundry equipment and installations are exceptionally good, hut to the
average foundry in which, as my experience goes, the facilities for really
good and scientific work are by no means what they might be. It would be
useless to put forward any propositions on the assumption that in every
foundry there exist the most modern furnace and apparatus and that every
foundry is equipped on the very latest lines of modern scientific foundry
practice. What is food to one founder might be poison to another, and there
is no way in which this could he better proved than by observing the results
in different foundries of mixing steel with iron in the cupola. Additions of
steel or other low carbon material to Cupola mixtures can only be successful
where, firstly, the Cupola is of the best design and the blower and air
connections of the proper size and arranged on the proper system, and
secondly, where the foreman and moulders are skilled in carrying large
bodies of metal almost immediately it is melted at very high temperature
from the Cupola to the moulding floor and have the necessary equipment in
the way of cranes, ladles, etc., to enable them so to do. The result of
adding steel to iron in foundries where the Cupola will not melt quick and
hot, and where perhaps there are not even blowing facilities for such
melting, and where there is no arrangement for disposing immediately of
fairly large bodies ol molten metal, is sure to be disappointing. A
temperature sufficiently high properly to melt and fluidise the steel is not
commanded and it will not mix thoroughly with the iron, and through slow
melting the steel will absorb great quantities of carbon and sulphur. This
means a patchy casting, hard and soft, weak and strong, and probably
containing more deleterious impurities than if nothing but iron had been
melted. I would like also to add, here, in relation to those cases of well
equipped and skilfully managed foundries, where mixtures of iron and steel
are used with success as regards the density, homogeneity and strength of
the castings produced, that such products will from the important point of
view of hardness and resistance to abrasion be inferior to the best Cast
Iron. Moreover, I do not think that, although tensile and transverse
strengths may be considerably raised they will be raised consistently above
the limits obtainable by iron alone unless the melting be done in an air
furnace or a crucible. My reasons, therefore, for rejecting the steel
addition method are these: that it is only practicable in the most
up-to-date and highly equipped foundries, and that it cannot give to certain
castings to the same degree as iron alone that quality ol hardness which mav
be described as resistance to wear and tear.
With reference to that class of mixtures which consist
wholly, or partly, of pig iron or pig iron and scrap re-melted, 1 condemn
that absolutely as a regular practice for these reasons. The Cupola furnace
is not in any sense of the word a purifying furnace, and should not be used
as such. It is certain that what the founder may achieve in lowering Silicon
by re-melting is at the cost of raising sulphur, and, furthermore, there
will be in a well-blown, quick-melting Cupola a considerable loss of a
valuable element, in which only too many foundry irons are seriously lacking
to begin with, Manganese. The object of re-melting is to reduce the Silicon
in the iron, and hence to close the grain. This object is achieved, but the
process is a costly one, and if we assume the cost of melting iron in a
Cupola to amount, all told, to 15/- per ton, it would seem distinctly
possible for the founder to pay an extra 15/- per ton for his pig, and
thereby obtain an iron of an analysis similar to that he expects to arrive
at by re-melting. The inverse relation between Silicon and Sulphur will
almost certainly be constantly exhibited in any Cast Iron melted in contact
with coke, but the smelter will have more skill and a greater choice of
method in bringing down a low silicon iron with a reasonable sulphur than
the ordinary Cupola hand will have, especially if you are willing to pay him
15/- a ton extra for his product. The alteration in the physical qualities
of Cast Iron by melting is the direct result of the changes in the chemical
composition due to melting, and these changes are almost impossible to
control accurately in a Cupola, and they will vary in their extent very
considerably with variations in the composition and arrangement of the fuel
or the iron charged, and with the oxidation to which the latter is exposed
during the heat and the duration of the whole process. It, therefore, seems
to me to be better to take originally pig iron of the Silicon and the
approximate density required holding a known Sulphur than to take a higher
Silicon pig than is necessary and melt it down twice, and this especially in
consideration of the manganese which will be eliminated, since if we scheme
to keep up the manganese in our metal we must begin with pig carrying very
high percentages of it, and such pig will be certainly very high in Carbon.
I therefore fall back on the old-fashioned system of mixing
pig irons of first smelting and scrap, and I shall venture to indicate
certain principles and methods by which our arrangement of such mixtures
should be governed. And, first of all, I should like to say a word against
that method which may be described as mixing, or grading, on the Silicon
basis. When, a generation ago, the influence of Silicon on the iron-carbide
system in Cast Iron was first discovered, it was clear that a most important
scientific discovery in connection with the properties of iron had been
made. It at once became apparent that the founder had been placed in
possession of a secret by which he could much more readily control the
hardness and softness of his castings than by any method previously known.
The result of this was that founders at once chose Silicon as a sole basis
for adjusting their mixtures and regarded it at once as the governing
element; and this consideration of Silicon has persisted almost universally
in foundries, and to my mind has brought the trade of iron founding in its
connection with high grade mechanical construction almost to the verge of
ruin. Silicon is certainly one of the governing elements in Cast Iron, but
by no means the only one, and with the knowledge that exists to-day
regarding the influence of the other elements, the effects of different
treatment and different temperatures, the infinitely extended understanding
of the forms in which carbon may be precipitated in iron, etc., it will be
granted that the arrangement ol iron mixture on a Silicon basis is
absolutely unscientific.
By this method many matters of equal importance are
overlooked. Surely an ironfounder called upon to make castings against a
difficult specification would do better to review the effects of the whole
of the foreign elements in his iron, to take into consideration how those
elements will affect and be affected by his melting, how they interact, and
how their presence or absence in certain quantities will govern the
condition and behaviour of his metal when it is tapped and poured. The most
fatal mistake that the ordinarily equipped founder can make when called upon
to turn out castings of high mechanical quality is to mix high and low
Silicon irons. It is against nature that such irons should melt together or
mix together. To bring up a percentage of hard, low Silicon iron with a
percentage of soft, high-Silicon iron, or to let down a percentage of soft,
high-Silicon iron with a percentage of hard, low-Silicon iron is not
“practical politics” as far as the average founder melting in the Cupola is
concerned. I am speaking now of fine castings, such as those referred to bv
me earlier on. The principal elements in Cast Iron are Silicon and Carbon,
and it will be found that the melting point and specific weight of pig iron
will be governed chiefly by these. I am almost prepared, then, to lay it
down as an axiom that to get a really fine casting, and get it regularly, we
must take nothing but irons for our mixture reasonably alike as regards
their Silicon and Carbon contents.
We shall as nearly as possible in all our irons fix our
Silicon and Carbon at the figure we wish them to stand at in the mixture
(and where we want strength and density these will both be very low), and we
shall control hardness, which practically means the final condition of the
carbon and its quantity, by careful adjustment of sulphur, manganese, and
phosphorus.
The second is, that we must be guided in the arrang-ement of
our mixture not only by the theoretical effect of certain percentage of
chemical elements on the finished casting, but also by the combined effect
of those elements on the physical phenomena of melting and cooling. It is in
the discovery of the correct balance between these two factors that I
consider the whole problem of iron-founding lies. Supposing we pursue the
chemical theory alone as applied to Cold Cast Iron, we shall be tempted to
take the purest possible pigs, especially as regards sulphur and phosphorus,
so that we may get a casting as nearly approximating steel in homogeneity as
possible. This is no doubt an attractive idea, but unfortunately we find
that it is not easy to carry out in practice, since our metal, when melted
by the Cupola, is lacking in fluidity, and, through want of heat and life,
cannot expel its gases, slags or oxides before solidification, the result
being blown or otherwise defective castings. It is useless, therefore, to
persist with what 1 may term the “ irreducible-minimum ” theory of
phosphorus and sulphur for ordinary Cupola-melted metal, though I quite
admit that the dangers mav be less in one foundry than in another, according
to the type and arrangement of the blow and the furnace and the skill of the
operators. It is therefore necessary, in order to get a casting free from
flaws and having a close and regular molecular structure throughout, to
introduce more of those elements into our iron that promote heat and widen
the range of fluidity of our metal, and we shall find that the elements most
useful in that direction are manganese and phosphorus. We shall find that
both of these may be raised to an astonishing degree, even where sulphur is
already high, without injuring strength, providing always that the melting
is properly done and that full advantage is taken in the casting operations
of the liveliness and fluidity of the metal yielded, and as long as the
graphitic Carbon in the casting is really low.
The gist of this principle is this: that the
final specific density of the casting will be the index of its mechanical
and physical qualities rather than its chemical analysis, within, of course,
reasonable limits, and this specific density is more easily achieved with a
hot fluid and lively metal holding fair quantities of phosphorus, manganese
and sulphur than with an ideal iron almost free from these elements. The
question might possibly be asked, if fluidity is the secret of success, will
not a high Silicon mixture give us that fluidity? The fluidity ofsilicious
iron is too well-established a fact to be traversed by me, but my own
practical experience is that the fluidity of Silicon, though very great
while it lasts, is narrow in range, and that the solidification of silicious iron
is more rapid once a certain amount of heat is lost than the solidification
of phosphoric or manganiferous metal. The best proof of this lies in the
well-known phenomena which we call liquid shrinkage in molten iron.
This liquid shrinkage or suction is certainly reduced to a minimum in high
Silicon mixtures, whereas all founders are familiar with the rapidity and
duration of it in strong- irons, and particularly with high manganese.
Personally, there is nothing 1 view with more satisfaction when my mould has
been filled with white-hot metal than the sinking of the metal in the
dead-head and feeders, providing I am satisfied that my moulding material is
sufficiently refractory and porous, and that the whole structure is properly
vented. I do not pretend here that I am uttering the pure milk of the
scientific world; I am merely mentioning matters of practical experience. I
do not understand the phenomena governing the fluidity of irons of different
chemical composition, but I do know that it is extremely difficult, if not
impossible, for me to get a tight casting of high specific weight with
silicious iron, however fluid, and that it is easy for me to get it with a
low silicon iron fairly high in manganese, sulphur and phosphorus.
Now you will have gathered that I hold that in order to get a
considerably improved Cast Iron such as is represented by the samples and
figures laid before you to-day, certain conditions are necessary.
The different irons in the mixture must all have a low
silicon.
They should, if possible, all have a low Carbon, but in any
case the final total carbon figure of the mixture must be low.
The mixture should be rich in Manganese and fairly rich in
phosphorus.
The Sulphur may stand at a higher figure than that generally
considered as the limit by the analyst.
The brands of pig iron which are most conveniently available
for my use for purposes of practical experiment are naturally those brands
belonging to the Company w ith whom I am associated, and these include
certain irons which, in different combinations, will give the desired result
for different castings. The actual mixture from which the results tabulated
were obtained is as follows :—
30% of FRODAIR Pig.
20% of BEARCLIFFE Pig.
25% of COLD BLAST Pig.
25% of Hard Selected Grey Scrap.
These irons are all smelted in England, and the following is
a rough description of them :—
FRODAIR is a dense iron carrying a low Silicon and a very low
Carbon, but melting very hot and fluid by reason of fairly high percentages
of phosphorus and manganese. Sulphur about ott%.
BEARCLIFFE is a tough iron of the true Hematite class
carrying about 1% of Silicon, a moderate Carbon, low phosphorus and high
manganese. Sulphur about 12%.
The COLD BLAST used is a coke iron with the typical Cold
Blast analysis, very low Carbon and Silicon, medium phosphorus, sulphur and
manganese.
SCRAP IRON used for the job was not analysed but taken on
trust. It was judged to hold low Silicon, medium carbon, high sulphur,
medium phosphorus and manganese.
In the mixture 30% of FRODAIR is taken to make absolutely
sure of the heat and fluidity of the metal and as a really low carbon basis
for the specification; 20% of BEARCLIFFE will keep phosphorus within safe
limits and will help fluidity by reason of its manganese; 25% of COLD BLAST
is now added to restore the carbon figure which has been raised somewhat by
the BEAR-CLI FEE, to keep sulphur within reasonable limits, and to guard
against extreme hardness. These last two irons give most of the strength and
toughness to the mixture. The scrap is used as an economy, and as much of it
might have l>een taken as the carbon and sulphur figure in the specification
would have allowed.
All the components have about the same Silicon. With these
irons mixed in this manner castings can be produced which would, I think,
answer many purposes for which steel to-day is used. Supposing for the
moment it be granted that this proposition is warranted and that it is open
to any founder present to use this kind of mixture and extend his business
in high grade castings for steam, hydraulic, pneumatic, oil, gas and other
engines and plant and other branches of mechanical constructions, let us for
a moment discuss the methods by which such a mixture can best be melted and
cast. The first essential point is, that the melting be done as rapidly and
as hot as possible. The great trouble in many foundries is that the Cupola
will not melt quickly and falls a good deal behind its scheduled capacity in
this respect. I would advise all those who are troubled in this way to
tackle their whole melting installation from the motor or engine to the
Cupola spout circumstantially, comprehensively and scientifically. The
failure wi generally he due to the blast, and not by any means to lack of
powrer or pressure, but probably, on the other hand, to too much of those,
the great bulk of which will be represented by inadequate tuverage and
resistance at the furnace and in the pipe connections. The plan I would
suggest to regulate this important matter is quite simple. Calculating at
35,000 ft. of air for each ton of iron melted, reckon what quantity of air
per minute the blower or fan should deliver at its outlet in accordance with
the scheduled caparity of the Cupola, that is to say, the weight of iron it
should melt within a given time, then see that you have a blowing machine so
devised as to deliver that quantity of air at that velocity which is
judged necessary to convey it freely into the furnace, presuming that
your pipes, wind box, and tuyeres are soregulated in shape and area as to
offer a negligible resistance to the action of the blower. This velocity
will varv somewhat with the size of the furnace i.e., with the distance
which the air has to travel after its first obstruction b} the charge. The
flow of air under pressure is a question very little understood in the
foundry, and most foremen would be astonished if they knew what vast
quantities of air will flow at high velocity through, say, 10-inch
unobstructed pipes under a very few ozs. of pressure. The foreman should fix
his pressure gauge on his blast-pipe just before it joins the fan casing,
and .this, I submit, is the only useful place in which a foundry gauge can
be placed. He then gets the indication he wants, i.e., the amount of air his
fan is delivering, because a given velocity is the result of a given
pressure and the volume is according to the velocity when the area of the
pipe is known. However, supposing we imagine that the air has free exit into
the furnace through the wind-box and tuyeres, there will still be some loss
of the effective work represented by the pressure at the fan through
friction in the connections, and this will vary according to the length of
the connections and their angles and bends. These latter are almost
impossible to reckon with accurately, and all I can say is, have no angles
or bends; but the loss by friction in a fairly straight pipe can be
estimated and can be provided for by a higher initial pressure, or by the
requisite increase in the size of the pipe in the direction of the Cupola.
The theory then is : let the blow^er deliver the right quantity of air at
its outlet, make sure that this air passes under all circumstances freely
into the Cupola, and this can only be done by having your total tuyere area
two or three times as great as the area of the blower outlet so that there
may be a veide margin for stoppages in front of the tuyeres throughout the
heat. It may be asked, how are we to know what quantity of air is delivered
by our blowers? Either you must find a firm supplying these instruments upon
whose statements you can absolutely rely, or vou must learn to calculate for
yourselves, and this latter is by far the best way. As regards Centrifugal
fans vou will find all the necessary instruction and information in
Kinealy’s book, set forth in a lucid, practical and fairly popular way, and
by studying the arguments and formula therein you will soon be enabled to
find what volume of air is represented by the pressures registered on your
gauge. Neither time nor the title of this lecture permits of my dealing with
the economical aspects of this question of blast supply. I have merely
mentioned the matter here because I believe it is at the bottom of nearly
all bad melting, and because, for the purposes of our improved Cast Iron, we
must have our mixture melted fast and melted hot.
Now, having brought down our mixture white hot we must get it
into the mould as nearly as possible at that heat, that is to say, we must
pour our metal as hot as ever we can get it. I do not believe in the dull
metal theory of casting. I suppose we could all quarrel very much about this
question of pouring temperature; metallurgists, experts, chemists, melters
and moulders are always discussing it and disagreeing about it. My own
experience is, the hotter the casting temperature the better the casting as
regards density and strength. If I am asked to say why, I can only offer a
humble opinion; the phenomena of cooling* iron are too uncertain and complex
to admit of more than this. I think that the hotter the iron the more the
impurities are held in solution, i.e., that as the process of cooling
proceeds molten iron throws out more dirt, or scum, or oxides. The hotter we
pour our iron the more rapid is the cooling- process in the mould, that is
to say, that the temperature will come down over a wider range in the same
time with hot than with dull metal, and hence the time for oxidation and
other re-actions is correspondingly reduced. Further, it must be remembered
that there is a vast molecular activity in molten metal, and the greater the
heat the greater that activity, the more complete the expulsion of gases. I
do not think there is any temperature in iron melted in a Cupola which
warrants a delay in pouring, and we must not look in this matter to
steel-founding* for a lesson Steel is melted and cast at much higher
temperatures than those attainable in the iron-foundry. Of course it is
understood that the hotter the iron the greater must be the care and the
wider the scope of venting, the more resistent and refractory the mould, and
the more ample and skilful the feeding.
The question may be put to me, in what practical way are
iron-founders going to benefit by supplying an improved Cast Iron? I w ill
answer that, to begin with, by another question. Has not the industry of
iron-founding suffered both loss and dignity through the continual rejection
of Cast Iron by engineers for all work where Specifications demand more than
the British Admiralty tests for transverse and tensile strengths. What I
claim is, that th*» potential strength and mechanical qualities of Cast Iron
are much higher than what is demanded by the severest .Specifications laid
down by modern engineers, and I argue from that that there is a big range of
castings now lost to Cast Iron which can be brought back into the foundry as
soon as the engineer can be convinced that higher Specifications can be met.
Let us look at this for a moment in a practical way and take the case of
cylindrical castings subject to internal pressure. For thin cylinders I take
the ordinary engineer’s formula, i.e., thickness equals diameter multiplied
by working pressure, divided by twice the safe working stress of the
material. Now if we take 9 tons as the tensile strength of Cast Iron and a
factor of safety of 6 (w'hich factor I wall adhere to throughout all cases)
we get a divisor in our formula of 3 tons. Mr. Pettigrew, in his book on the
locomotive (a standard work) reduces this to 3,000 lbs. i.e., under 2½ tons,
so he apparently w ill not trust Cast Iron even to have a 9 ton tensile
strength. However, we stick to our 3 tons, and taking a steam cylinder of
16-in. diameter and a pressure of 130 lbs., we find we require a wall
thickness of 5/14 of an inch. But supposing our Cast Iron will stand a 13
ton tensile test we then, by the same formula, get a necessary wall
thickness of 3/14 of an inch—a saving of 2/sths in our material. For engine
cylinders the thickness against bursting stress is, of course, largely
reinforced by extra thickness against piston abrasion; our improved Cast
Iron will have much better wearing qualities as well as much higher tensile
strength, and the engineer will soon find he can also considerably cut down
his allowance for re-boring. Take a hydraulic cylinder with a diameter of 8
inches and a pressure of 1,500 lbs. per square inch. For this class of
construction, where the thickness of the metal will be great in proportion
to the bore, we require another formula, since allowance must be made for
the diminished stress on the outer layers of the cylinder wall. I use a
formula kindly worked out for me after impartial consideration of the whole
problem by an engineering friend of mine, Mr. Leslie Sadler, of Bradford.
With Admiralty Cast Iron we get a wall thickness of 2.47 ins., with our
improved Cast Iron we reduce this to 1.27 in., practically half. The section
would, of course, be less with a good mild steel, but I submit that where an
engineer might reject a section of, say, 2½ ins. in Cast Iron, he might
adopt one of, say, 1^ in preference to steel.
It is these direct and concrete considerations which lead me
to urge founders to re-adopt the tensile test for Cast Iron. The transverse
test is not a direct indication of the tensile strength. It is at the best
only a comparative test, and is only of value in that respect in testing
similar types of iron. The difficulty of the even grip and pull on the
tensile test-piece has been overcome in the best laboratories, and the
tensile test on the round bar is a much more reliable index of the quality
of Cast Iron than the load test on a square bar. The square bar is a bad
shape, its angles offending the most rudimentary principles of design in
Cast Iron constructions. And further, and perhaps most important of all, it
will be found that a high tensile record will never accompany that kind of
hardness which means trouble in machining, so that this test will always
keep the founder on absolutely safe ground when his castings get through
into the fitting shop. Beyond the question of raised strength and decreased
section and weight in castings there lie other possibilities. It is
impossible for me to give a number of concrete cases; let it suffice to say
that it is within our powers to issue castings of much harder and denser and
more elastic material than the engineer is accustomed to receive from the
iron foundry. By increased strength and density, and, above all, by greater
regularity of quality and skill in manufacture, many of those strains and
stresses and erosive, mordant and abrasive actions which it is the tendency
of our age to meet by Steel may be met by Cast Iron. On the occasion of a
recent visit to Norway—a country now making very great advances in the
engineering industry—I was discussing the question of the size and section
of iron screw propellers with a local firm of shipbuilders. My friend was
complaining, and quite justly, of the great bulk and ungraceful shape of
these and deploring the fact that the larger they were the more ugly and
unscientific they looked. I ascertained that the Norwegian Lloyds prescribe
beyond certain limits of dimension an extra half-inch of section of the
blade shoulder for every extra 6 inches of diameter of the screw. The
tensile test of the metal is only 8 tons. My proposition was, that for each
succeeding- increase of 6 ins. in diameter there should be an extra ton of
tensile strength in the iron, the section at the shoulder remaining the same
I was asked to approach the Norwegian Lloyds with this suggestion. I did so,
and it was accepted. Later 1 saw some propellers made by my. friend to the
new specification, and the improvement in line and in driving efficiency was
quite wonderful. Here was a case in which iron was getting a fair chance.
The old method was simply a long step towards the abandonment of iron for
bronze, because in the larger screw's the shape soon became so ugly and the
bulk so enormous that the engineer would not have them at all; by the new
method the iron founder was encouraged to improve his material and keep a
hold on the orders.
Perhaps 1 am offering a counsel of perfection, and 1 admit Ido
not have to live by the profits of ironfounding—perhaps 1 am running my head
up against a brick wall, still I venture to ask why not, instead of wildly
competing in the direction of cheapness and ol the substitution of soulless
mechanism for intelligent handicraft, compete to turn out a better iron to
meet the advancing specifications of to-day; an iron reinforced against
water-hammer in pipes and valves, against back-lash in gears, against the
growth induced by variations of temperature, against the shock of the hammer
and the fret of the piston, against the bite of acid and the sweat of heat.
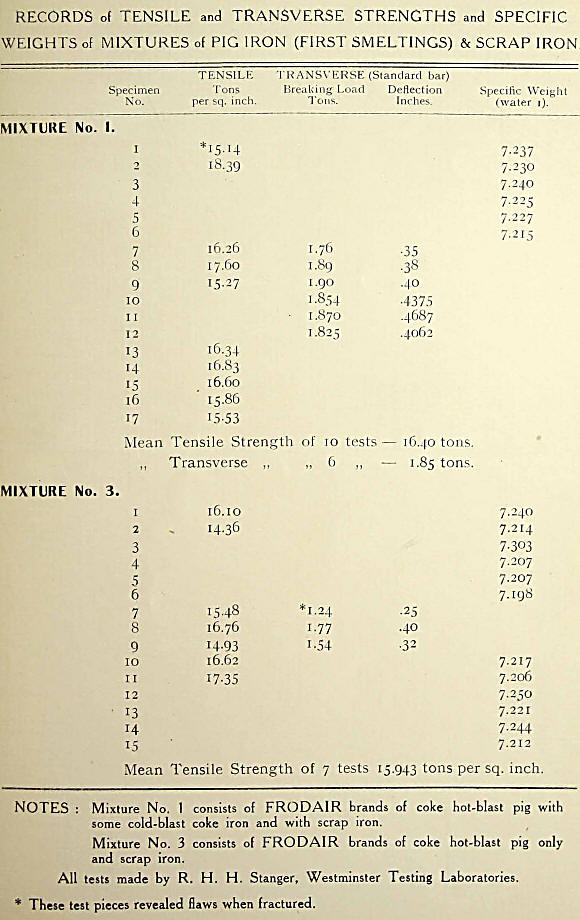 |