MANUAL OF AGRICULTURE,
INCLUDING THE APPLICATION THERETO OF CHEMISTRY, GEOLOGY, BOTANY, ANIMAL
PHYSIOLOGY, AND METEOROLOGY.
By Richard Henderson,
Coldstream, Berwickshire.
[Premium—Twenty-Five Sovereigns.]
Chapter I.—Introduction.
Agriculture, literally,
tillage of the ground, is both a science and an art: a science, in so far
as its principles are co-extensive with those of chemistry and the cognate
physical sciences; an art, in the intelligent direction of these
principles to the practical end of best developing the food-producing
properties of the soil. The importance of founding the practice of this
art in this country upon a more thorough and widely diffused knowledge of
its scientific principles will be granted, when it is stated on the best
authority, that by a generally thorough cultivation of the soil the annual
agricultural products of Great Britain might be doubled in quantity. And
it is a fact, that we annually import food from other countries to the
value of L.80,000,000 sterling, which fact may, undoubtedly, increase
commerce and beget the comity of nations; but at the same time it might
leave us in a hazardous position in the event of a sudden political
emergency. Agriculture is the oldest of the arts; for we may rest assured
that Adam delved, however problematical may be the question whether "Eve
span." Amongst the ancient Egyptians, and later, under the Roman Empire,
its practice attained a high measure of success, but it rested on a merely
empirical basis. Not before the present century has any general scientific
knowledge of the laws of nature, which regulate the art, characterised its
numerous professors.
Whatever may be the
varieties of soil and climate—and these, together with the subsidiary
circumstances of available human labour and of markets, may be said to
determine the particular mode of agriculture suitable for any
locality,—the great fundamental laws, in conformity with which alone is
truly successful practice possible, are comprised in the physical sciences
following:—viz., Chemistry, Botany, Geology, Animal Physiology, and
Meteorology. The last, to reverse the order, under the simple name of
"weather," is a subject of interest, scientific or otherwise, to every
farmer. It teaches a system of forecast of weather changes. Forewarning is
forearming; and by adjusting farm operations accordingly, great loss is
avoided. Animal Physiology treats of the bodily structure and the
functions of the bodily organs of our domesticated animals; and in that
department of it we earn the general treatment best fitted to ensure their
healthy procreation and profitable development. Geology has to do with the
formation and nature of the Earth's crust, the forces which have been at
work in preparing it for its present condition, and those at present
affecting its modification. In its relation to agriculture, it reveals to
the farmer the various compositions of soils, and their derivation, and it
gives him practical hints upon drainage operations. Botany, in its bearing
upon agriculture, teaches the systematic classification of the various
plants scattered over the face of the globe, their native localities, the
variety of soil and climate best suited to the cultivation and growth of
individual plants, and their internal structure, and modes of reproduction
and growth. Chemistry—the grammar, so to speak, of all the physical
sciences—acquaints us with the primary original materials of earth, air,
and water, and consequently of all animal and vegetable life. As being the
most fundamental of all the physical sciences bearing upon agriculture,
its consideration in that relation comes naturally first.
Chapter II.—Of Chemistry.
Our earth, with its sea and
atmosphere, and all whatsover therein contained, is composed of about
sixty-three original and simple elements, whose substance cannot be
further reduced. Of these only four occur in the atmosphere; upwards of
thirty have been found in sea water; whilst in the solid structure of the
globe, the whole are to be found in varying proportions. They can exist in
three states, viz., solid, liquid, or gaseous; [Liquids are technically
known as incompressible fluids, and gasses as compressible fluids.] and
that either alone or in combination. And such changes of state take place
at fixed degrees of heat or temperature for each. The several elements
consist of an aggregation of atoms, those of each particular elements
being always alike in material weight and volume. In the gaseous state,
and whilst free from combination, the atoms of all the elements are of
equal volume; but they vary in weight, and also, of course, in point of
material. Although not in an apparent manner, the elements can, under
certain conditions, be artificially reduced to their atomic state—i.e.,
isolation of individual atoms—by the aid of heat or electricity. When thus
reduced to the free, or as it is called, the nascent state, the atoms of
any element have a tendency to unite with those of one or more different
elements, when brought into contact with the latter. This is being
continually effected through the agency of natural means; and, as will be
seen, it is the cause of all physical change on our planet. The atoms of
one element unite with those of another in certain fixed invariable
proportions, and they have a greater tendency to unite with, or affinity
for, those of some elements than of others. What is known as chemical
combination is this union of the atoms of different elements, and the
result is a compound body or substance. The least number of atoms in such
an union, or rather, the least quantity of such a compound body, that can
be formed, or exist in a free state, is called a molecule; wherefore,
chemical compounds are composed of an aggregation of molecules. Still more
complicated chemical compounds are formed by the union of the molecules of
different compound bodies. The proportion of molecules requisite with a
given quantity of different molecules, to form a new compound, is called
the equivalent of the latter. In all chemical action, heat is given off or
taken up; in the process of combination, it is evolved, in that of
separation, its absorption is requisite thereto. A certain amount of heat
is absorbed by all substances while they pass from the solid to the
liquid, and from the liquid to the gaseous forms, which heat remains in
abeyance — latent, until such substances are again transformed into their
original forms, and then it is evolved. When the majority of chemical
bodies assume the solid, instead of the liquid or gaseous form, they
appear as small particles of a definite geometrical shape, called
crystals, each compound invariably preserving its own peculiarly
distinctive crystaline form. Moreover, whatever be the size of any united
accumulation of specific crystals, the aggregate mass shapes itself into
the geometrical type of its minutest constituent crystal. Chemical bodies
not observing this law, in the process of their solidification, assume a
structureless texture, and are called amorphous, literally, without form.
Again, numerous compounds arising through the agency of animal and plant
life, show neither a crystaline nor an amorphous, but a cellular or
organised texture. A chemical combination of elements is something quite
distinct from mixture pure and simple —that is to say, mere mechanical
union. In the latter case, there is no interchange of the several
atoms,—no chemical action takes place. There is merely mechanical
juxtaposition of particles. By way of illustration, take the preparation
of common mortar. There, before the addition of water to the lime shells,
the latter are in the state called caustic lime, or oxide of calcium—a
combination of the simple metallic element calcium, and the simple gaseous
element oxygen—one atom of the one in chemical combination with one atom
of the other; whence its technical symbol in chemistry Ca. O. Upon the
addition of water, a violent disturbance in the mass occurs, together with
the evolution of much heat. And this action continues until the certain
amount of water requisite to enter into combination with all the caustic
lime present has been added. Any amount of water superadded thereto, and
the sand, are simply mixed with it mechanically. No further immediate
chemical action ensues.
Another illustration of chemical combination
is the common class-room experiment, showing the composition of water,
wherein the two gases oxygen and hydrogen are mixed in the proper
proportions in a vessel. Still there is but mechanical union till a flame
is applied, when the two gases instantaneously explode with violence, and
the chemical combination will be found to have produced an entirely new
body, a liquid-water.
The elements are, according to their
possession of certain physical properties, arbitrarily divided into two
classes—the metals and the non-metals. And those, again, which are met
with in the combination present in animal and vegetable life, are further
classified into two groups, viz., organic and inorganic.
The organic, in all substances, are capable of
being separated from the inorganic, and driven off in the shape of gases,
by simple combustion. The inorganic always remain, as the ashes of the
substance consumed. In the organic elements life, animal or vegetable, may
be said to have had its seat.
The terms, however, have another
application,—"organic" being used in the case of those complicated
compounds whereof the element carbon is an invariable constituent;
"inorganic," in that of the simpler compounds, with fewer atoms composing
their molecules. Chemical compounds, according to their marked
characteristics, are all classified under three divisions, viz., acids,
bases, and salts. The first two exhibit quite different properties; but
when, under certain conditions, they are brought into contact, they lose
their distinguishing properties and unite to form the neutral compounds of
the third division.
Particular acids are stronger than others, and can, so to speak, expel the
weaker—those acids which have a less degree of affinity for the base—from
the salts, and occupy the place in their stead. From a limited point of
view, the most characteristic acids may be said to possess a sour taste,
and the property of turning a solution of blue litmus to a red colour;
whilst, on the other hand, the most marked bases, such as potash, soda,
ammonia, and lime—alkalies so called—can restore the solution of blue
litmus thus reddened to its original colour, and they have a peculiar
soapy taste. To the chemist, however, the terms "acids" and "base" imply
the possession of properties of a much wider and less limited description.
Out of all the chemical elements, about 18
only are discover- . able in the blood and tissues of man and the lower
animals, and in the juices and fibres of plants. Hence, these should exist
in greater or less quantity in our cultivated soils, seeing that animal
life depends primarily upon vegetable life, and that the latter again
derives its main sustenance directly from the soil.
Of these 18, none exist in the free state, but
as various compounds, in bodies animal and vegetable. They are:—
1st. Organic, comprising Oxygen, Hydrogen,
Nitrogen, and Carbon; and
2d. Inorganic, comprising Silicon, Aluminium, Potassium, Sodium, Calcium,
Magnesium, Phosphorus, Sulphur, Iron, Manganese, Chlorine, Bromine,
Iodine, and Fluorine.
Oxygen.—Of all the elements, oxygen occurs the
most abundantly throughout nature. It exists free in the atmosphere, of
its total bulk contributing 1-5th part. In combination with other
elements, it constitutes one-half the weight of the solid globe, and
8-9ths of that of water. It is an invisible gas, tasteless, and without
smell. Excepting fluorine, it enters into combination with all the
elements. In this process, called oxidation, heat is always, light
sometimes, evolved. Flame consists of gas in a high state of ignition,
caused by the oxidation of the substance consumed. During combustion, new
chemical compounds, chiefly gaseous, are being formed, but no element is
annihilated.; Animals inhale oxygen into their lungs, where it oxidises
certain elements in the blood and tissues, and thus keeps up the degree of
warmth necessary for life. Oxidation is much more rapid in undiluted
oxygen than in the atmosphere.
Hydrogen is another invisible gas, devoid of
taste and smell. Its principal combination is with oxygen, in the form of
water—two atoms of hydrogen to one of oxygen,—whence its chemical symbol H2O.
Hydrogen is the lightest of all the elements, and it is taken as the unit
with which to compare the others, The symbolical letters, it may be
remarked, representing the different elements, represent also their
combining weights, or the weight of their respective atoms compared with
hydrogen's. An atom, or any volume of oxygen, is 16 times the weight of an
atom or equal volume of hydrogen; and as water is composed of two parts of
hydrogen to one of oxygen, the latter constitutes 8-9ths of the weight and
a third part of the volume of water. Hydrogen has been found free in
sundry volcanic gases, and it can be obtained from the decomposition of
water, through the agency of certain metals. Water enters into combination
with many substances, and in so doing, in almost every instance, one of
the atoms of hydrogen, in the molecule of water, is replaced by some
equivalent in the compound into which it enters, and free hydrogen is
given off. Nitrogen
is also an invisible, inodorous, tasteless gas. It exists free in the
atmosphere, mixed with oxygen, forming about 4-5ths of the bulk of
atmospheric air. It is a most inert element, incapable of entering into
direct combination with any other except oxygen, and even then with
difficulty, and only by means of the electric spark. By very indirect
processes, however, it enters into important combinations with hydrogen as
well as with oxygen. It forms five several oxides, the principal one of
which is a combination of 5 atoms of oxygen with 2 of nitrogen —N2O5,
which, combined with a molecule of water, constitutes nitric acid, H2O
+ N2O5 = 2 (HNO3). With hydrogen, it
forms ammonia—1 atom of nitrogen to 3 of hydrogen—NH3.
Carbon we meet with free and as a solid in
three distinct forms, physically different, but possessing in common the
same chemical properties. These are:—(a.) The precious diamond; (b.)
graphite or plumbago, popularly known as black lead; and (c.) charcoal. It
is found neither as a fluid nor a gas in the free state. It is present in
all organised structures. It forms about 50 per cent. of the residue of
plant life when the latter is charred, and access of atmospheric air or
oxygen prevented, for oxidised carbon escapes as a gas. It enters into
exceedingly complicated compounds, the consideration of which forms a
special branch of chemical science, called organic chemistry. Combined
with oxygen, it forms carbonic acid CO2—an invisible, ponderous
gas. Plant life is
unable to assimilate these organic elements in their free state, but only
when they exist in combination with certain other elements. Such compounds
are water, nitric acid, ammonia, and carbonic acid. In the organised
structures of plants these compounds are broken up or resolved, and their
constituent parts economised in the building up of new organisations,
which in their turn are metamorphosed for the structure of animal life.
The atmosphere and soil, but chiefly the latter, are the media through
which these elements are rendered available for the necessities of plant
life. Silicon.—Next
directing the attention to the inorganic elements, it is to be remarked
that silicon, next to oxygen, is the most abundant element in nature. It
does not occur free, but as an oxide, SiO2, known as silicic or
silica acid. In that state it is nearly pure, under the forms of quartz,
flint, and sand. Silica, though in a variable quantity, is always present
in the ashes of plants. Chiefly is it plentiful in cereals and grasses. It
forms the hard glistening surface of straw and bamboo. In most plants,
however, it rarely exceeds 5 or 6 per cent. of the residual ash.
Aluminium is a bright lustrous metal of
excessive lightness. It does not occur free, but as an oxide—A12O3.
There is but a slight trace of it in the ashes of plants, although in
combination with silica, under the name of silicate of alumina, it forms
the basis of the clay of our soil. From an agricultural point of view, it
is therefore of importance.
Potassium, when with difficulty prepared free,
is a light metal of silvery appearance, and so soft as easily to be cut
with a knife. Thrown into water, it decomposes it. One atom of potassium
replaces one atom of hydrogen, and heat is evolved in sufficient quantity
to ignite the liberated hydrogen. It rapidly absorbs oxygen from the
atmosphere, forming the oxide K2O—Kalium being the technical
name given to potassium in chemistry. This oxide has a powerful affinity
for water. The combination is potash KHO. The change can be represented by
a chemical equation as follows:—K2O + H2O=2(KHO).
One molecule of oxide of potassium and one of water form two molcules of
potash. It is one of the most important compounds in the ash of plants,
forming from 20 to 50 per cent. of its weight. It is mainly present in
roots and tubers, seeds and grasses, and in the leaves and branches of
trees. Sodium.—Sodium
is a metal closely resembling potassium in all its features. These two,
together with four other less important elements, which do not enter into
living structures, are called the metals of the alkalies. They decompose
water at all temperatures, and combining violently with oxygen, they form
powerful caustic and alkaline basic oxides, which possess a strong
affinity for water, which last cannot be expelled from them by heat agency
alone. The principal oxide of sodium is Na2O— Natrium = sodium.
This combined with water is HNaO, or soda. The compounds of sodium are
widely distributed, and along with those of potassium abound in the
primary rocks, as well as in sea-water. Soda is a less important
constituent, and forms a less proportion of the ash of plants than potash.
It is more largely present in the ash of marine than of land plants.
Calcium.—-Calcium, when free, is a light
yellow metal. Readily combining with the oxygen of the atmosphere, it
becomes the oxide CaO,—lime. Lime has a strong affinity for water, and
decomposes it at any temperature. It forms with water CaOH2O,
or slaked lime. Calcium compounds largely from the rock-forming materials
of the globe, in such varieties as, e.g., chalk and limestone. From the
last, lime for ordinary use is prepared, by driving off, by means of heat,
the carbonic acid,— limestone being a salt called carbonate of lime,
composed of carbonic acid and lime as a base. Lime discharges most
important functions in the soil, in the way of breaking up compounds,
liberating their constituents in such a manner, as to render them readily
available for the purposes of plant life. Its percentage in plant ash,
varies as much as from 1 to 40.
Magnesium is a silvery white metal. If
strongly heated, it takes fire in- the air, burning with a dazzling white
light, and forming the oxide MgO, known as magnesia. In dry air it does
not oxidise. It is slowly acted upon by cold water, more rapidly by hot.
As the carbonate of magnesia, it occurs, together with carbonate of lime,
in enormous quantity in the species of limestone called dolomite. It most
abounds in the ash of grains, contributing 12 or 13 per cent. of the same.
In the ash of the remaining parts of cereals and of other plants, it
varies from 2 to 4 per cent.
Phosphorus does not occur free in nature, but
is generally to be found combined with oxygen and calcium. When prepared
free, it is a yellowish semi-transparent, and waxy solid. It is
exceedingly inflammable and oxidizable. It ignites on the slightest
friction, whence chemists only keep it with safety under water. In the air
it readily oxidizes, giving off white fumes, and in the dark emitting a
pale lambent light. If slowly oxidized, its white fumes are the oxide
P203. Upon its ignition the resulting oxide is P205,—phosphoric acid.
Phosphoric acid may be considered as the most important inorganic
constituent of plant life. Seeds have it in larger quantity, as it
constitutes about 30 per cent. of the residual ash of grains. It is
equally important in animal life, being a most essential constituent of
the brain, nerves, blood, and bones.
Sulphur is found free in nature as yellow
crystals. It is found combined too, with many metals, forming sulphides,
—which are the ores from which the several metals are usually obtained.
Again, combined with oxygen in addition to the metals, it forms the salts
called sulphates. Sulphur, during the process of ignition, produces the
oxide SO2, a colourless gas, soon intimating its presence,
however, by inducing the sense of suffocation. SO3 is its
principal oxide; which, combined with a molecule of water, is H2SO4, the
important sulphuric acid. The amount of sulphur found in the ash of plants
is inconsiderable, —over 1 or 2 per cent.
Iron.—Of the invaluable element iron, the
appearance and main properties are presumably known to all. It is rarely
met with naturally pure, save in the form of meteoric stones; but in the
well-known form of wrought iron it is nearly quite pure. Although for
minga very small percentage of plant ash, it is still most essential, and
to animal life, as well as plant, several parts of animal bodies demanding
it in abundance for their constitution. It forms three classes of oxides,
the presence of two of which in the soil is of moment. These are (a) the
ferrous, or proto-oxide, Fe2O2, and (6) the ferric,
or per-oxide Fe2O3. As the symbol shows, the ferrous
has less oxygen in combination; but when exposed to the atmosphere, it
greedily. absorbs the additional amount of oxygen, which will constitute
it the ferric oxide. Inasmuch as the latter, again, is easily divorced
from the oxygen, in contact with other combinations in the soil, iron, it
will be seen, discharges valuable functions there, as an oxygen
contributor. It is iron also which imparts the variety of colour to the
different classes of soil.
Manganese, prepared free, is a reddish-white,
brittle, excessively hard metal, which the slightest exposure to the air
oxidises. The oxide produced is MnO. MnO2, another of its
oxides, is much used in the labortory for producing oxygen. The quantity
of this element in plant life is very minute.
Chlorine is not found naturally free; but when
produced free, it is a greenish yellow gas, pungently odorous, and most
irritably injurious to the mucuous membrane. For hydrogen it has a strong
affinity, the combination forming hydrochloric acid. Combining with the
metals, salts are produced by it, called chlorides, of which the principal
is chloride of sodium, or common table salt.
Bromine, Iodine, and Fluorine all resemble
chlorine in their respective qualities, the four forming a detached group
in chemical science. Bromine and iodine are almost entirely confined to
sea-water and marine plants. Traces of fluorine are found in the blood,
teeth, and bones.
Copper.—Minute traces of copper have been found in the ashes of animal and
vegetable organisations, but it is not considered a necessary element in
the economy of animal and plant life.
The absorption, accordingly, of these
inorganic or "mineral " constituents by plants, is entirely effected from
the soil, by means of their roots. Such constituents are present in the
soil in many various combinations, some of them being almost insoluble in
water. Rain-water, however, dissolves carbonic acid from the atmospheric
air, and water containing it in solution, can dissolve compounds insoluble
in it whilst pure and simple. Carbonate and phosphate of lime are thus
rendered available for plant nutrition. It is believed that some indirect
power is exercised by plant roots themselves, in breaking up the insoluble
compounds in the soil.
The different combinations in the soil
affording the necessary inorganic elements to plant life, and the various
functions of the latter to whose operation such combinations are
subjected, fall to be discussed in a subsequent chapter.
Chapter III.—Of Geology.
The student, in having his attention turned to
the science of geology, cannot fail to be struck with the vastness of the
field which is there opened out to research; and when he encounters
undeniable proofs of our globe having endured through countless ages ere
it became fitted to receive its present species of inhabitants, he more
distinctly can realise the hopeless incomprehensibility of the word
eternity. Whatever
the source of the sixty-three original elements, the greatest physicists
are of opinion that when these became united in the mass, resulting in an
independent planet, ruled by the sun's attraction, such a degree of heat
must have prevailed therein, as to cause such elements to exist in the
gaseous state. As the heat would depart by radiation into proximate space,
the denser compounds would tend to unite as a congeries, so that when the
earth had commenced its career of revolution around the sun, its
consistence would be that of a pasty mass, enveloped with dense vapours
and gases. Its present spheroidal shape would ensue upon its rapid
revolution upon its own axis. With the process of cooling down would keep
pace that of the condensation of its surrounding fogs and gases into air
and water, whilst its more solid mass would concentrate in bulk, pressing
inwards towards the centre of gravity with the attendant effect of
irregularity of surface, caused by the absence of a uniform degree of
internal resistance to such external pressure. With the increased
intensity of this pressure, more marked would become mountain and valley.
Moreover, the resisting force of the internally confined fluid substance
would gradually prevail over the weaker portions of the crust, and its
upheaval, with all the violence of earthquake and volcanic convulsion,
would vastly exaggerate the superficial irregularity of Moses' " dry
land." Thus did it appear above the face of the waters, but naked as
yet,—sterile, without soil, entirely devoid of living organisation. Then
gradually atmospheric action would crumble down those bare rocks exposed
to its action. The detritus washed into the surrounding depth of waters,
there subjected to the superincumbent pressure for epochs of time, became
solid strata or layers, thence to be upheaved and exposed to the
atmospheric process, as a rock-forming material different in nature and
texture from its originators. And so on, the alternate depression and
upheaval continues even to the present time, but in an infinitesimally
less degree, for the cover of vegetation over the larger portion of the
earth's surface protects from the erosive action of the atmosphere, and
earthquakes are less frequent, and the more considerable volcanoes for the
most part inert. By the earth's crust is meant the thickness of it which
has come under the cognisance of geologists, and it bears an inappreciable
proportion, of course, to the earth's diameter. Heat increases in the
ratio of our depth of penetration through the crust, proving the immensity
of heat still present within our globe, which is conjectured internally to
be in a molten state, or at least in a honey-combed condition, with molten
matter filling the cells. This is evidenced by the phenomena of lava and
hot springs, even more strikingly than by the large increase of
temperature in deep artificial mines. The rocks, —and this term includes
clay, gravel, and sand deposits,—which compose this crust, are divided
into—1st, The igneous, being those formed by the agency of fire, or from
fused melted matter, and preserving their original condition; 2d, The
aqueous or sedimentary, comprising such as have been formed by the deposit
of detritus of rocks exposed to the air, and laid down under the water in
regular strata; 3d, The aerial, or such deposits as have been accumulated
by atmospheric agencies. Sand dunes, calcareous sands compacted by rain,
the debris at the bottom of cliffs, and soil are examples; 4th, The
metamorphic, those which have undergone change of texture since the
eruption or deposit of their constituents. Traces of organised remains
begin to be met with in the earliest aqueous rocks, and thus attain a
higher development in proportion to our ascent to the latest aqueous
deposits,— stratifications,—till it becomes perfect as that of the living
forms, both animal and vegetable, now existing. The branch of geology
dealing with such traces, or fossils, as they are called, testifying to
the forms of life co-existent with the deposition of the specific
materials of the strata where they are found, is named Palaeontology—an
abstruse study, its prosecution demanding the preliminary of a highly
scientific training.
The igneous rocks principally exist as granite and trap. They are chemical
productions, i.e., have been consolidated from fusion by chemical means.
Besides forming the solid framework of the earth, and the foundation of
the other rocks, they are upheaved, and constitute the principal mountain
chains, and they are exposed in masses of enormous area. They are also
poured out in profusion as lava and scoriae during volcanic action, and
they permeate the rents and crevices of the sedimentary rocks. All igneous
rocks are composed of minerals, silicates, to wit, i.e., salts formed by
the union of silicic acid with a base. These silicates are divided into
two classes—silicates of magnesia and silicates of alumina ; and the
various subdivisions in each are constituted by so many mixtures with
silicates of potash, soda, lime, iron, manganese, &c. The uncovered masses
of igneous rock generally being situated at high altitudes, the soils they
form are at such an elevation as to be incapable of cultivation, and they
are accordingly left in the natural condition. But when such soils exist
in practicable situations for the agriculturist, or the detritus of them
is conveyed thither, great fertility obtains, and the soil is easily
worked. This is specially predicable of soils derived from trap rocks.
Metamorphic rocks are produced by the
alterations effected by heat in the texture and structure, and by its
rearrangement of the atoms of the constituents of their originators.
Although resulting from the changes in strata of all epochs, still they,
for the most part, lie over or against the huge igneous masses, being
comprehended in the so-called Laurentian, Cambrian, and Silurian eras of
formation. Together with the igneous, they constitute the principal part
of wilder Wales and the Scotch Highlands; and whilst such tracts admit of
little cultivation, they are admirably suited for sheep runs.
The stratified rocks have been produced
mechanically, as we have seen, by the agency of the atmosphere and water;
chemically by the precipitation of their constituents from solution in
water, e.g., rock salt; and organically by the agency of organised living
structures, e.g., coal and peat, both of which are the remains of plant
life, and limestone, the remains of minute animalculae. Stratified rocks
have a threefold classification, denoting the epochs of their respective
formations, viz, the Primary or Palaeozoic—ancient; Secondary or
Mesozoic—middle; and Tertiary or Cainozoic—modern. The expression primary;
signifies no fixed era or standpoint of time,—merely that indefinite
portion of the past when the first sedimentary rocks began to be deposited
beneath the water. By " era," too, the geologist understands any period
comprehending groups of living organisms bearing points of close
resemblance to each other. The names given to the different formations
have generally been taken from that of the most characteristic or useful
rock in the group, which may sometimes include a stratum of quite an
opposite texture and composition. Thus the old red sandstone formation
includes some of the densest clay rocks, and it may appear contradictory
to apply the term sandstone, clearly suitable in one district to the clay
slate of another. But as these two contain the identical class of fossils,
their similarity of age is demonstrated, and hence the justice of their
sharing the same family appellation.
The stratified rocks are arranged in the
following leading groups, in order of time:—
I. Those of the Primary or Palaeozoic period,
including (a.) The
Laurentian or Pre-Cambrian era rocks, which are principally composed of
gneiss, a metamorphosed granite, whose original granite particles have
been disintegrated and redeposited, and compacted with a different
structure and texture.
(b.) Cambrian era rocks, composed of grits, slates, and conglomerates.
(c.) Silurian era, divided into (1) lower, comprising the Lin-gula
Llandeilo, and Caradoc beds, and (2) the upper division, comprising the
Llandovery, Wenlock, and Ludlow beds.
(d.) Devonian and Old Bed Sandstone era, comprising the lower, middle, and
upper Devonian beds of England, and the like three Old Bed Sandstone beds
of Scotland.
(e.) Carboniferous.—Carboniferous or mountain limestone, millstone grit,
and the coal measures.
(f.) Permian—the lower, containing red marl, sandstone, and conglomerate,
and upper containing lower and upper mag-nesian limestone.
II. Secondary or Mesozoic period, containing
(a.) Triassic, or New Bed Sandstone, of lower,
middle, and upper formations.
(b.) Jurassic era, embracing Lias (lower, middle, and upper); Oolite,
lower—inferior oolite, fullers' earth, great or Bath oolite, forest
marble; middle—Oxford clay, coralline oolite; and the upper—Kimmeridge
clay, Portland and Purbeck beds.
(c.) Cretaceous era—Hastings sand, Weald clay, lower green-sand, gault,
upper greensand, chalk marls, and chalk.
III. Tertiary or Cainozoic
period, including
(a.) Eocene era—lower, middle, and upper,—the lower including plastic and
London clays, and the middle and upper the deposits formed in estuaries.
(b.) Miocene era—Lignites and leaf-beds.
(c.) Pliocene era—Crag formations.
(d) Pleistocene or post-tertiary era, comprising boulder clay or glacial
drift, raised sea beaches, fens, peat bogs, river deltas, alluvium, sand
dunes, and so on.
These main groups, and, with a few exceptions, the various strata they
respectively comprise are all represented in the British Islands,—an
evidence of the extensive convulsions they have undergone.
Although in few countries do they observe such
an unbroken series, still they invariably observe the cardinal order of
deposition, whatever hiatus may occur in it. Periodic convulsion
throughout immense areas is evidenced by the existence of fossils of land
plants, which flourished on the soil of certain strata, being discovered
beneath immense stratification of a different class. To the variety of the
British rock formations are due the multiplicity of its types of natural
scenery, its many different modes of agricultural practice—all included,
too, within so small a superficies. This variation in practice is a
consequence of the variety of soil, which, as a rule, has a direct
relation to its underlying rock formation. By the term "soil" is
understood so much of the surface as in cultivated ground comes under the
operation of the plough, and which in land still in a state of nature
would come under such influence were it to be cultivated: "subsoil" is
what comes immediately under it. Where identity of chemical constituents
does not exist between the soil and its subjacent rock formation, the
constituents of the former have been imported from another source. But in
all cases, rock and subsoil alike have an important bearing upon the
questions of facility of drainage, the physical features of exposure,
flatness, or declivity of the soil.
As already mentioned, the soils of the
Laurentian, Cambrian, and Silurian eras are mostly found at a high
elevation; and, in addition, being for most part of a poor description,
are seldom cultivated with any degree of success. On the other hand, those
of the Devonian and its companion series possess all degrees of value.
In the Carboniferous era, the soils of the
coal measures groups are inferior, and generally much neglected; those of
the millstone grit are also poor and thin. In the mountain limestone
group, they are classed as of medium quality; and, as in Derbyshire", they
afford good pasture ground. In the Permian, the soils are of a light, dry
description, and easily cultivable. As the name implies, most of its
limestone holds too much magnesia for agricultural purposes. Of the
Triassic era, the soils are variable. They are kept under pasturage, over
the marls, and are then good for dairy purposes. Above the sandstones they
are deep and dry, although not of high quality. In the Jurassic, they
range from the densest quality, such as, e.g., Lias, Oxford, and Bradford
stiff clays, to that of a thin light sandy type. Excepting those above the
chalk marls, the gaults and wealden clays, the Cretaceous affords soils of
a light dry nature, which produce, under pasturage, an excellent herbage,
sweet and nutritious, and well adapted for sheep stock. Coming to the
Eocene era, we find such dense soils as the London clay, with others of a
lighter description in immediate contact with them. In the Pliocene, we
encounter the soils typified by the rich alluvium of river-side
deposits—the "carse", lands of Scotland, deltas; and also meet fens,
peat-mosses, and land reclaimed Dutch-wise, from the sea.
In districts where the subsoil is deep, and a
considerable space intervenes between the upper soil and its underlying
rocks, there is generally a scarcity of stones for building or road-making
purposes; and this is especially the case in clayey formations, and those
of the Pleistocene era. The clay, however, can be burnt into bricks, and
material in substitution of road "metal"—which latter, however, is a poor
make-shift for stone. The nature of the subjacent rocky formations too has
considerable influence upon the question of water supply. Thus the
numerous fissures in the chalk and oolite formations act as natural main
drains throughout large areas, intensifying the droughts of hot summers.
The opposite extreme is reached when rocks or subsoils are of a close or
retentive description, unfavourable to the percolation of water. Hence it
is that a knowledge of the position and nature of the subsoil and
underlying rocks is essential in the conduct of extensive drainage works.
The term "dip" means the inclination of strata to the earth's centre, and
is measured by the angle formed by the intersection of the plane of the
horizon with the plane of the beds themselves. "Strike" means a line at
right angles to the dip. "Outcrop " is where the beds appear at the
surface. By rock "structure" is meant the peculiar arrangement of its
component parts in the mass, e.g., stratified or columnar structure (like
that of the igneous rocks of Staffa and the Giant's Causeway.) "Texture"
implies the minute arrangement of the composing particles; and
"compositions" applies to their chemical features. "Joints" are the lines
of fracture seen dividing rock masses into separate lumps or blocks, and
which facilitate the quarrying of them. "Faults" are such fractures of the
strata series as raise or depress the level of the strata on one side of
such faults above or below that of the strata on their other side, and
thus break the continuity of stratification.
Chapter IV.—Of Botany.
Of the science of Botany, physiological botany
is that one of its departments which most concerns the practical
agriculturist, treating, as it does, of the different organs of plants,
and their respective functions. The classification of plant life is a
field of study too extensive for his time and opportunities. But as all
the British cultivated plants are included in a very few "orders," the
comparative slightness of their physiological variations renders his
acquisition of this branch so much the easier by its approximation to a
uniform applicability.
A normally developed plant consists of four
different organs, viz., root, stem, leaves, and flowers,—the first three
being nutritive organs, and the fourth that of reproduction. They are
alike modifications of one structure, for the fundamental structure of all
plant forms is the simple cell. Cells are minute, round, bladder-like
vessels, which cohere and form cellular tissue, named parenchyma. They
have their origin in a thin mucilaginous compound called protoplasm, which
is considered the seat of life. A small germ, termed a nucleus, appears in
the protoplasm, which presently, with some of the protoplasm, gets
enclosed by a species of sac or covering, and this constitutes a cell.
Increasing, the nucleus seems to be divided, and the cell-wall closing
round either portion, forms two distinct cells. And so on indefinitely.
This cell-wall or envelope is formed of a substance termed cellulose, the
composition of which will be subsequently given. Active cells, besides
containing protoplasm and nuclei, for the purposes of increase, and also
their several characteristic contents, have, when situated at the exterior
portions of plants, chlorophyll as well, which is their green colouring
matter, and has the property, when it is acted upon by sunlight, of
assimilating certain elements from the atmosphere. The shells of nuts and
other seeds are composed of hard solidified cells; the roots of turnips,
potatoes, &c, almost entirely of juicy cells. The cell-walls or coverings
of different plant groups have characteristic marks, whether dotted,
barred, reticulated, or with spirals or other quaint devices. Cells cohere
by means of connective tissue, supposed to be secreted from their walls. "
Intercellular" canals are the spaces formed where cells do not adhere on
all sides ; and they serve for circulating air through the plant
structure. Fibres and vessels are formed by the modification of simple
cells. The former appear to be formed of elongated cells, which have been
filled up with woody substances. These firmly cohering, form woody fibre.
The cells of vessels are not thickened or filled up. They are formed by
strings of cells, so to speak, having their contiguous partitions
destroyed, so as to form a continuous tube or channel. They possess the
distinctive markings of the cells, whence they are derivative. Their
office would appear to be that of promoting not sap but air circulation in
plants. Cells constitute the entire formation of some plants, such as
fungi, mosses, and sea plants; and these are termed cellular. They have no
flowers, and propagate by means of cellular germs. The remaining plants
are called vascular, as they contain vessels, fibres, and cells. Excepting
the fern tribes, they have flowers more or less conspicuous, and they are
reproduced by the instrumentality of true seeds.
A cellular skin or covering, called the
epidermis, extends over every part of the plant. It is divided into the
cuticle or outer portion, and the derma or inner portion. The cells
composing it are colourless, but through them shines the chlorophyll
contained in the cells underneath. When examined by aid of the microscope,
on its surface are seen oval-shaped organs with small openings in their
centres leading through the epidermis into air chambers. These openings
are called stomata, and serve for the purposes of perspiration and
exhalation of liquids and gases. They are found on all parts of the plant
above ground, excepting the petals or coloured portions of the flower, and
they seem to possess the power of opening and closing according to the
moist or dry condition of the atmosphere. The epidermal cells get modified
to assume the form of hairs and scales, as seen on leaves and other parts
of certain plants.
Selecting the root as the first organ for consideration, the student must
make its acquaintance as the "descending axis" of botanists. It is the
seed's primary development, and always has a downward direction. This
delicate process branches into numerous fibrils, whose number and
dimensions rapidly increase. "Radicle hairs" are scattered over them, and
through these and the cells of the more delicate parts of the fibrils'
epidermis is absorbed the plant's nourishment from the soil. The
elongation of roots proceeding from their extremities, they are fitted to
penetrate in every direction in quest of suitable food. This organ's
development assumes a great diversity of form. Of species, we have
terrestrial roots, such as have been already described,—though it may be
remarked that the roots of fungi, as in the case of sea-weeds, merely
serve for anchors, the fungus obtaining its food from the atmosphere;
aquatic roots, belonging to floating plants, which are unattached,
floating freely in the water, and absorbing nutrition from that medium,
e.g., duck weed; aerial, as in the family of orchids, which in the tropics
are attached to foliage, and their roots hang in the air, whose moisture
they absorb, and against the branches, whence they derive food from the
tree's decay; and parasitic, those fastened to the substance of other
plants whose sap they absorb, and they have no direct connection with the
soil. Such are moulds, the dodder, injurious to clover, and the festive
mistletoe. The root
functions accordingly are, with exceptions to fix the plant, to absorb
nourishment from the soil, and occasionally, as in the turnip, to serve as
a magazine of nourishment for the plant's use in promoting its growth at a
future season. The absorbent cells of roots would appear to possess a
power of selection and rejection over suitable and injurious food
constituents. The absorbtive process is considered to be that of endomosis,
which signifies the property of gases and fluids, enabling them to pass
through certain membranes in order to mingle with other fluids and gases
possessing different densities and compositions. "Exosmosis" expresses the
converse process. The
stem is the organ which bears the leaves and flowers; and, like the root,
it assumes all possible phases of modification. Some stems are long,
others short, so as to be scarcely seen above ground; some burrow to a
varied extent under ground; whilst others again, for support, have to
cling to stronger neighbours; familiar specimens, whereof respectively are
ordinary trees and grain plants, the turnip, the quicken grass and potato,
and ivy. The tuber of the potato is in fact a stem, its eyes the buds,
producing branches and leaves. Stems are divided into three great classes,
viz., exogenous, endogenous, and acrogenous. A stem of the first of these
divisions increases in diameter by the addition of matter to its outer
circumference. A cross section shows in its centre the pith, with lines
radiating from it to the circumference or bark, which are called medullary
rays, and concentric rings round the pith, each marking a year's growth.
Outermost is the bark, easily separable from the wood proper. The annual
increase to wood and bark takes place immediately under the latter in a
layer of slimy substance termed the cambium. The outer and newer wood is
called the laburnum, the inner or heart-wood, which is denser, generally
of a darker colour, and through which there is less sap circulation, is
named the duramen. The bark has three layers,—the innermost tough and
fibrous, forming in some plants, e.g., flax, "the bast."
An endogenous stem increases in diameter by
the collection in its cellular centre of bundles of fibres and vessels,
which swell out and extend the outer circumference. In a cross section we
see no pith, no concentric rings, no true separable bark, but on the
contrary, a hardened cellular mass of bundles of vessels and an internally
hard inseparable bark. These fascicles of fibres run from the bark inwards
and downwards towards the stem's centre, effecting a firmly interlaced
structure. The
increase of an acrogenous stem takes place at its summit, as exemplified
in the case of ferns and tree-ferns. The whole length is of nearly the
same diameter, it is marked on the outside by the scars of leaves, whose
bases, indeed, compose it. In cross section there appears a cellular mass,
often hollow in the centre, with bundles of vessels interspersed
throughout. Stems produce buds, or branches bearing them ; in some cases
only at their extremities, "terminal" buds, in whose destruction is
involved the death of the plant; in other cases, both terminal and
lateral. Some buds, instead of developing into branches, become modified
into thorns. Both branches and thorns have a continuity of the central
stem-substance, and are thus distinguished from such prickles as, e.g.,
the briars, which are merely developments of the epidermis, having no
direct connection with the stem. The functions of the stem are chiefly to
support the leaves and flowers, and to afford them a due exposure to the
influence of sun and air. The modifications of leaf form are endless.
Microscopically examined, the leaf epidermis shows numerous hairs and
stomata. Immediately beneath it are discovered elongated or "palisaded"
cells, having a close vertical arrangement; and spaces are numerously
interspersed, corresponding with the stomata above. Inferiorly occur other
cells more freely and openly arranged, with the fibres and vessels
constituting the veins of the leaf running through them. The arrangement
of leaf-veins— the venation of leaves—affords another means of plant
classification. Along with exogenous stems, plants have a reticulated
venation:—with endogenous, a herring-bone, venation, or the veins running
parallel from the central vein to the leaf's margin. The petiole or
leaf-stalk attaches it to the stem. On any ordinary tree it is more or
less round and fibrous; in the rhubarb plant again, it is thick and juicy,
and constitutes the edible part. Sometimes it has almost the identical
functions of the leaf itself; in sundry pines there is no distinction. The
functions of the leaf are occasionally assumed by the stipules—small
leaflet-like bodies at the base of the petiole, and very apparent in rose,
pansy, and clover plants. Frequently they form tendrils and sheaths of the
petiole. The midrib or central main vein of the leaf is a continuation of
the petiole. Leaves
are either simple or compound,—the former when the petiole carries but one
blade, and has no joint above the point of union with the stem. Such are
the leaves of the oak or beech trees. Compound leaves' have their blades
subdivided into separate distinct lengths, each of them being articulated
to the petiole, as in the case of the horse-chestnut. Leaf margins may be
entire, serrated, crenate, and so on; the blades, according to the apex,
acute, obtuse, &c. When divided laterally from margin to midrib they cleft
pinnately; longitudinally, they are pal-mately cleft, and so forth.
Vernation is the varied mode of the folding up
of young leaves in the bud. The attachment of leaves to stem is spirally
arranged in a strict mathematical order. There is the like analogy between
branches and stems, rootlets and root. Certain plants lose their leaves
annually, others retain them permanently. Of the first division, the
leaves of some wither and fall away on the completion of bud formation.
Such plants are called deciduous, as oak and ash trees. Of others, the
leaves wither and decay, but still adhere, as do those of lilies. Plants
of the second division retain their leaves of one season's growth till the
full development of their successors in the next; the majority of our
evergreens for example. When their functions have been nearly discharged,
leaves change their colour, and from the secretion of inorganic matter in
their cells, they shrivel up. Simultaneously, a constriction of the base
of the petiole becomes gradually complete, whereupon all the cells of
stalk and leaf die, and the latter falls to the ground.
Leaf functions are analogous to those of the
lungs. Leaves seem to expose plant sap to the action of air and light,
which frees their juices from excessive moisture, and. induces such
chemical changes of their substance as elaborate them into suitable
compounds for assimilation by the plant, to the end that in all its parts
cell-building may multiply.
In certain plants the leaves are possessed of
strange supplementary powers. For instance, the leaf of Venus's fly-trap
has the property of curling inwards and enfolding the luckless insect
which may have alighted thereon. And stranger still, this duress is
effected from a carnivorous propensity; for physiologists declare that the
plant thereupon absorbs the juices of the insect for its own nourishment.
In the pitcher plant some of the leaves act as watertight reservoirs, by
assuming the form and direction best suited for receiving the supply of
moisture; and frequently they contain a considerable supply of water. The
large quantity of fluid containing solid and gaseous bodies in solution,
and absorbed by the delicate cells of roots, has an upward current through
the central portion of the stem, and reaching the leaves, where it
undergoes the changes adverted to, it next takes a downward current
through the interior parts of the stem, delivering growth materials
through its course.
The belief at one time was general of the excretory power of roots over
plant waste and matter injurious to its health; but it has been
surrendered by contemporary physiologists. The absorbent and exhalent
power of plants over moisture has, it will be seen, an important influence
upon the passage of water from soil to atmosphere, when the immense extent
of forest area throughout the world is considered. Much of it, however, is
retransferred to the ground, having been condensed on the colder leaf
surface by warmer air currents. The wholesale hewing down of forests has
been observed to produce a scarcity of rain in regions where no such
privation existed while the forests flourished. Plants by their green
colouring matter act as purifiers of the air by absorbing carbonic acid,
so hurtful in excess to animal life. This process is accomplished by such
colouring matter when subjected to solar action, decomposing the acid, and
whilst freeing the oxygen, assimilating the carbon.
A flower when normally developed consists of
four parts—two called the enveloping organs, viz., the calyx or outer
circle, and the corolla or coloured portion, and the remaining two called
the essential organs, as being necessary for the production of seed, and
named respectively the stamens, and in the centre the pistil. All parts
alike are modifications of the leaf. The leaves forming the calyx are
named sepals; those of the corolla, petals ; and in each they occur,
either united or separate, and assume infinity of shape. The stamen
consists of a stalk or filament, frequently so short as to make it appear
absent, which supports two bags called anther lobes, these containing a
dust powder—the pollen of the flower, necessary for the fertilising of the
ovules or germs of the embryo, and these are held by the pistil or ovary.
Above this last is the style or stalk, having at its extremity the stigma,
upon which the pollen must be deposited ere it can come into contact with
the ovules. The changes in the bean flower may be taken to illustrate the
stages of the reproductive process. The grains of pollen on the stigma
extend minute processes down the style into the ovary, where, coming into
contact with the ovules, fertilisation ensues. Next the calyx, corolla,
and stamens, having performed their functions, wither and die. The pistil
or ovary is now disclosed, as having assumed the shape of a pod, and
within it the fertilised ovules have developed into a row of beans or
seeds. The plants of some varieties have staminate or male flowers on some
of them; pistillate or feminine flowers on others Others bear both sexes
on one and the same plant; whilst the generality bear flowers containing
both stamen and pistil together, and these are called perfect flowers. The
various modes of fertilisation in plants is an interesting study; and the
unlikely agencies through which the access of pollen to the ovules is
effected bear evidence of the highest design. Insects have a great share
in this office, through the adherent pollen on their legs and bodies
getting deposited on the stigmata of the successive flowers upon which
they alight. Indeed, it is maintained by the most eminent savants that the
primary office of the many bright hues, varied scents, and tasted
secretions of flowers, is to allure the visitation of the insect tribe for
the purpose of pollen transportation. The wind also carries many kinds of
pollen dust to its due destination.
To enter upon the consideration of the
countless different conformation of parts, relation as to numbers and
modes of arrangement of the flower, would be to transgress the limits of
this manual. The
seed, then, is the fertilised matured ovule. It contains, along with a new
plant in embryo, a supply of nutriment for its sustenance when it begins
to germinate, and before it can derive that from the soil direct. The
embryo consists of the radicle, or root rudiment, the seed leaves or lobes
called cotyledons, and the plumule or young stem. The number of cotyledons
affords yet another standard of plant classification. Exogenous stemmed
plants have two cotyledons in their seeds, and hence are called
dicotyledonous; endogenous, only one, and are therefore styled mono-cotyledonous;
whilst flowerless plants which have no true seeds, and consequently no
cotyledons, are named acotyledons. In the pea and bean the supply of
nutrition is incorporated with the cotyledons; in grains it is quite
distinct and separate from them. In the former the cotyledons remain
beneath the surface, and are absorbed by the radicle and plumule. From the
turnip seed the two cotyledons spring above ground, appearing as two
smooth leaves, and only decaying when the rough leaves proper sprout and
develop. It is with these tender cotyledons that the turnip beetle or
"fly" works such havoc, consuming them and thereby arresting the seed
functions. All grasses and grains are monocoty-ledonous. The principal
food ingredients stored up with the embryo in the seeds are starch and
nitrogenous and phosphatic compounds. The absence of direct light and the
presence of air and moisture are necessary for the germination of the
seed. The air and moisture are requisite for effecting chemical changes
essential to germination; the latter softens the seed constituents, and
with the oxygen in contact the atoms change places, and soluble compounds
are formed. Those are absorbed by the cells of the embryo, its several
processes are developed, and the rudiments of root and stem produced.
The complicated bodies,—called the proximate
constituents,— elaborated and organised by plant life from the simple
inorganic compounds derived from soil and atmosphere, are classified into
three divisions, according to the different kinds of nutrition these
subserve in the animal body. The three divisions are as follows: the
Amylaceous or Saccharine, Oleaginous, and the Albuminous. The first group
is entirely composed of carbon, hydrogen, and oxygen, the two last
entering in the exact proportions requisite to form water, whence they are
often termed carbo-hydrates. In Roscoe's Chemistry they are arranged under
the heads of Sucroses, C12H22O11,
represented by sucrose or cane sugar; Glucoses, C6H12O6,
represented by dextrose or grape sugar; and Amyloses, C6H10O5,
represented by dextrin, starch, cellulose, and gum. The amyloses are
insoluble in water, but the action of certain acids converts them into
dextrose, which is soluble in water; although not to the same extent as is
sucrose. Gum and cellulose are with difficulty converted into dextrose;
starch, less so. Starch, however, soon assumes a soluble form under the
action of the saliva and other juices of the body. The action of the
organic compound diastase, which is always found present in seeds
beginning to germinate, renders starch stored up in the seed soluble for
the use of the embryo at that stage. The starch first assumes the
properties of dextrin, and then it is readily changed to dextrose. It will
be noticed that the addition of one molecule of water to those of the
third division will make them assume the same formula as those of the
second. The
composition of the oleaginous compounds is the same, but with the amount
of hydrogen much in excess of the proportion necessary, with the contained
oxygen to form water. Consequently, they are styled hydro-carbons.
Glycerin, C3H8O3, is the base of all
fatty compounds, which vary with the different proportions of acids in
combination with it. Most fats and oils contain a mixture of all these.
The three principal acids are— palmitic, C16H32O2;
oleic, C18H34O2; and stearic, C18H36O2.
The albuminous compounds contain nitrogen,
carbon, hydrogen, oxygen, phosphorus, and sulphur; they are also called
the nitrogenous compounds. The composition of them all varies but little;
and they are convertible by a slight rearrangement of atoms. These
compounds are derivable by animals only from vegetable sources. They are
assimilated without undergoing much alteration; and in the animal
constitution have almost the identical composition as the relative
compounds in plant life. The following table shows the composition of the
principal members of this group which are met with in the animal body:—
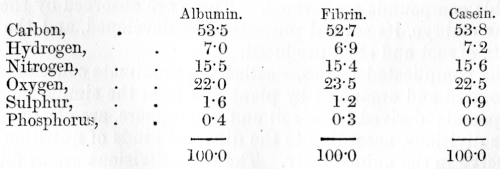
Albumin and fibrin abound in blood and muscle.
The glutin of wheat corresponds to fibrin; and albumin is found in the
juices and seeds of plants. Casein is the albuminous compound altered the
functional action of these plants that in their first season's growth they
do not develop flowers and seed, but store up a sufficient supply of
nourishment in the hypertrophied parts for the basis of flower and seed
growth during the following season. The rape and cauliflower plants are
likewise descendants of nearly related stock: the abnormal development
being seated in the leaf-stalks and leaves of the former, and in the
flower-stalks of the latter. Varieties are producible by means of the
artificial fertilisation of the seed of one plant through the application
of pollen taken from the flower of some other particularly developed plant
of the same species. In this way has been produced the countless varieties
of wheat, barley, oats, and other cultivated plants. Hybrids can also be
produced by fertilising the ovules of one species with pollen from the
flower of another species. But, in common with the hybrids of the animal
kingdom, these are incapable of reproduction.
All the grains and grasses of our annual crops
belong to the order Gramineae, which is one of the class Endogenae;
wherefore they are all endogenous stemmed, and their seed embryo is mono-cotyledonous.
Wheat forms the genus Triticum; barley, Hordeum; oats, Avena; rye-grass,
Lolium; and so on.
These genera are respectively subdivided into several species ; these
again into innumerable varieties. The bean, pea, and clover plants belong
to the order Leguminosae, of the class Exogense; and have therefore
exogenous stems, and are dicotyledonous. The bean plant constitutes the
genus Faba; the pea, Pisum; and the clover, Trifolium. To the class
Exogenae also belong the turnip, rape, cabbage, kohl-rabi, and wild
mustard plants, which with others constitute the order Cruciferae, with
its genera, species, and varieties respectively.
Chapter V.—Of Animal Physiology.
There are many striking points of analogy
between animal and vegetable physiology. In point of fact, when we look at
the elementary organisations in each great natural division, the boundary
line between them is difficult to be drawn; and the forms are numerous,
regarding which it is matter of debate as to which great division they
properly belong. As we ascend to more highly-developed forms in either,
the line of demarcation becomes more readily definable. The highest forms
of plant life possess no nervous system, no cavity for the reception and
digestion of solid food, in other words, no stomach ; and they have no
independent power of locomotion ; all of which qualities, on the other
hadd, belong to the higher forms of animal life. Another cardinal
distinction is that whilst plants can assimilate the elements necessary
for building up and maintaining their structrue from such simple or
inorganic compounds as carbonic acid, ammonia, and nitric acid, animal
bodies, on the other hand can derive them from such complex organised
compounds only as are formed by plants out of the simpler elements. This
prepares us for the important fact, that all the actions of animal life
consist in the liberation of heat or force attendant upon the
disorganisation of the organic compounds forming the tissues.
Consequently, every movement of the animal implies a consumption or using
up of materials in its frame. Muscular action is the contraction and
expansion of the delicate fibres composing muscle structure in obedience
to nervous stimulus; and such contraction is caused by a liberation of
atoms or molecules, and the resulting disorganisation or breaking up into
simple compounds of the proximate substances composing the muscle fibres.
Plants prepare their proximate constituents from the simple inorganic
compounds by sun heat and light agency; whilst animals derive their
possible existence from the liberation of latent force when these
organised compounds are broken up. Plants also absorb carbonic acid and
give off oxygen; animals inhale oxygen and exhale carbonic acid.
Nevertheless there are to be met with in the
lowest scale of development forms of animal life devoid of stomach,
nervous system, and independent locomotion; and also vegetable forms
endowed with some degree of locomotion, and organs functionally resembling
the stomach, and which do not obey the plant laws of exhaling pure oxygen
and subsistence upon inorganic compounds.
As in the vegetable, so in the animal kingdom
is it with regard to the fundamentally structural nature of the simple
cell, created from a nucleus and the organic compound protoplasm by that
mysterious agency hitherto only known and defined as "vital force."
The functions treated of in animal physiology
come under the three heads of Nutrition, Reproduction, and Correlation,
which last includes the consideration of the functions of sense and
motion, or those by which the total organism is brought into relation with
external nature.
Beginning in order, we find in all the more highly-developed classes an
alimentary canal, into which is received food material, undergoing those
processes which render it fit for assimilation. Then it is passed along
the tortuous channel, where its nutritive elements are absorbed and thence
conveyed to the blood, and at whose extremity the residuum of indigestible
matter is excreted. The solid food when received into the mouth is broken
up by the teeth, and mingled with saliva during mastication; and thus
rendered into a pulpy mass easy to be swallowed, and prepared for
stomachic action. Besides softening the food the saliva exerts certain
chemical influences upon it; notably converting insoluble amylaceous
bodies into soluble saccharine bodies. The mass passes through the gullet,
entering the stomach at the cardiac orifice. There it is acted upon by
several secretions, the principal of these being the gastric juice, whose
properties closely resemble those of hydrochloric acid. When the various
compounds are nearly dissolved, they are passed on through the pyloric
orifice of the stomach to the intestines in the condition called chyme.
Chyme is a pasty substance, containing dissolved saccharine matter and
undissolved starch, albuminous bodies broken up and wholly or partially
dissolved, oleaginous bodies broken up but undissolved, such solid
indigestible portions as have been enacted upon by the gastric fluids, and
some of the liquids swallowed along with the solid food. The intestines,
according to their diameter, are divided into the large and small.
Continuing from the stomach, the small intestine is nominally
distinguished as the duodenum, jejunum and ileum; and the large intestine
as the caecum, colon, and rectum. The latter distinction is less merely
nominal, the rectum being less puckered or convoluted than the other two.
At the union of large and small intestines occurs the ileo-caecal valve,
allowing a passage but one way from the small to the large.
Ere the chyme has entered far into the
duodenum it is subjected to the action of the intestinal juices organised
in various glands. Chief are the bile and pancreatic juices, secreted by
the liver and pancreas respectively. These serve further to dissolve the
albuminous compounds, of emulsifying or saponifying the oily constituents
of the chyme, and of recommencing the conversion process of starch into
sugar, which had been arrested by the gastric fluids. And thereby all are
alike rendered capable of direct absorption. The chyme continuing its
course through the intestines, has its available compounds absorbed and
carried to the blood, and the insoluble, indigestible residue voided from
the rectum. At this
stage it may be as well, before adverting to the processes of absorption
and nutrition, to consider the constitution and circulation of the blood.
Like the sap of plants in its grand work of
supplying all the animal tissues with the necessary food for health and
maintenance, it has to discharge the additional functions of keeping up
the animal temperature in every part, and of removing waste tissue
substance and matter deleterious to life. Actually it consists of a
colourless fluid containing innumerable minute globules,—"corpuscles,"—the
greater part of which are red in colour, and give the blood its
characteristic hue. Its fluid portion, the liquor sanguinis, is composed
of the "serum," holding fibrin and other compounds in solution. The fibrin
exposed to the atmosphere has a tendency to coagulate, whence blood clot.
In the first stage of coagulation its total constituents appear as one
jelly-looking mass, but in a little the serum oozes thence as a yellowish
slimy fluid. The corpuscles, however, remain contained in the fibrin. The
serum contains about 8 per cent. of albumen, and with the exception of the
fibrin and the corpuscles, the whole constituents of the blood. Roscoe
gives the following graphic formula as the average composition of the
blood:—
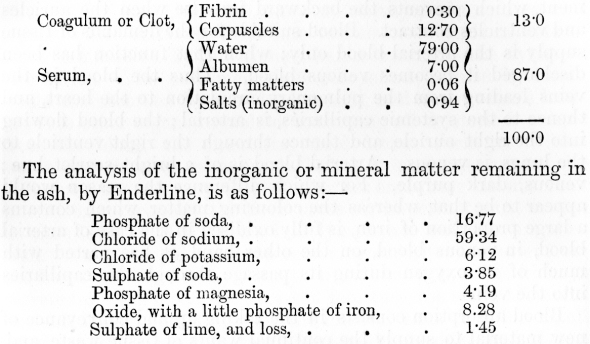
The heart, by its continual alternate muscular
contraction and expansion, keeps up an uninterrupted circulation of the
blood through the whole animal frame. There are four cavities in the
heart, two auricles and two ventricles. At either side of the heart
respectively are an auricle and a ventricle. The auricle of the left side
opens directly into the ventricle of the same side. And so is it at the
heart's right side. But the whole course of the circulation intervenes
between right and left auricles and ventricles respectively. The
contraction then of the left auricle filled with blood forces it into the
corresponding ventricle, which, at the same time, expands in order to
receive it. Next, the contraction of the left ventricle throws its
contents into the main arteries, forcing it along them into all their
branches throughout the body, and into their capillaries as well—the
minute vessels closely interlaced, which permeate all the corporeal
tissues. From these delicate tubes the blood enters the veins, and through
them is forced back to enter the heart's right auricle; whereupon is
completed the systemic circulation, or that whereby every part of the body
receives an unfailing constant supply of nutritive blood.
As in the former instance, the right auricle
pumps the blood into the right ventricle, whence it enters the pulmonary
artery, and through it the lungs. In the lungs it is exposed to the action
of the atmospheric air which they inhale, and having undergone the
consequent important change of constitution it passes next into veins
communicating directly with the left auricle, having thus completed the
pulmonary circulation. The vessels leading from the ventricles are
arteries; into the auricles, veins. Both orders of vessels are connected
by means of the delicate capillaries so as to form a continuous channel.
The blood is kept circulating in one direction by means of the valvular
arrangement, which prevents the backward impulse when the auricles and
ventricles contract. Blood suitable for the demands of tissue supply is
the arterial blood only; when that function has been discharged it becomes
venous blood. Thus the blood in the veins leading from the pulmonary
circulation to the heart, and thence to the systemic capillaries, is
arterial; the blood flowing into the right auricle, and thence through the
right ventricle to the lungs, is venous. Arterial blood is of a bright
scarlet hue; venous, dark purple. For which difference the reason would
appear to be that, whereas the colouring matter, which contains a large
proportion of iron, is fully oxidised in the case of arterial blood, in
venous blood, on the other hand, it has parted with much of its oxygen
during its passage through the capillaries into the veins.
Blood absorption consists in the taking up and
conveyance of new material to supply the continual wants of tissue waste,
and, in addition, the removal of this waste, which otherwise would effect
the destruction of the parts throwing it off. The other absorbents besides
the blood-vessels are the lacteals and lymphatics. The lacteals are
confined to the intestinal canal; the lymphatics are distributed through
all parts of the body where the presence of blood-vessels occurs. Both
alike are connected with and pass through the lymphatic glands, which are
supposed to have the power of further organising the compounds absorbed by
the vessels. The absorption of substances received into the alimentary
canal is effected by the blood-vessels and lacteals. Blood-vessels absorb
all soluble matter, whether albuminous or saccharine, &c, directly through
the enclosing membranes of the vessels. Hence, fluids and matter in
solution can be absorbed by the blood-vessels before the passage of food
from the stomach. The other two sets appear to have an affinity for
particular substances. The lacteals chiefly absorb oleaginous substances,
and their contents are then called chyle. In the intestines are numerous
processes called villi, consisting of a network of minute blood vessels
surrounding one or more lacteals, and all covered with mucous membrane
like the other portions of the intestinal canal; and here it is that
absorption principally takes place, although it does so more or less at
all parts, even to the canal's extremity. It is still matter of doubt and
conjecture as to the exact source whence the lymphatics directly absorb
their contents. They are supposed to absorb the excess of liquor sanguinis
effused for nutritive purposes by the delicate capillaries into the
tissues, and also such compounds resulting from tissue changes as are not
totally excrementitious, but capable of further utilisation and
absorption, after they may have been more highly organised and again
carried into nutritive circulation, The nutritive substances being almost
entirely absorbed by vessels leading into the veins, they are conveyed
through several organs, which elaborate them into various compounds
capable of being assimilated by the tissues before reaching the systemic
circulation. The excrementitious compounds formed by tissue waste are
absorbed by the blood-vessels, and these vessels leading into other organs
capable of extracting all deleterious matter from the blood, the
circulating fluid leaves them freed from waste and noxious compounds
before it again permeates the different parts of the system. In further
organising the absorbed nutritious compounds, the liver takes an important
part, as also do the glands of the lacteals and lymphatics and the lungs
as well. The principal agents in removing superfluous matter from the
blood are the kidneys, lungs, liver, and skin. The kidneys secrete from
the blood the excess of moisture, the waste albuminous substances, and
nearly all the mineral salts in solution, all of which are conveyed from
them to the bladder, and thence voided as urine. In the lungs the venous
blood gets exposed to the oxygen there inhaled in the common air, and such
blood greedily absorbs the oxygen. Carbon and hydrogen combine with it,
and are exhaled with the expired breath as carbonic acid and watery vapour.
The oxygen combines with the blood's colouring matter as well, and is
readily yielded up by the latter to the tissues, in order that there may
be effected the oxidisation and chemical change of their component
substances. Besides its functions in the process of digestion and of
organising compounds absorbed by the blood, the liver has to discharge the
supplementary one as well of purifying the blood from the presence of
certain bodies, which are for the most part compounds of carbon and
hydrogen. The skin excretes gases and moisture—carbonic acid and watery
vapour for the most part; but also minute quantities of compounds similar
to those in urine, and this in the acts of sensible and insensible
perspiration alike. Such compounds as those last mentioned are also to
some extent exhaled from the lungs.
The principal bodily excretions are urine and
the faeces, which, committed to the soil, are still of value for the
purposes of plant life. Urine, as we have seen, chiefly contains
disorganised albuminous compounds and inorganic salts. Summarily, the
different compounds of faeces may be stated to be those which have been
taken into the body with the food and carried through it, without having
been assimilated. Unless there is consumption of food rich in albuminous
matter, or the latter is present in the former in an indigestible state,
the faeces contain but a small proportion of nitrogen. The principal
constituents are unassimilated inorganic salts.
To epitomise, alimentary substances are
introduced into the stomach, there to be broken up and dissolved, and
passed thence into the intestines, where they are still more completely
fitted for assimilation by the action of the absorbents. The saliva
changes insoluble starchy matters into soluble saccharine compounds, until
this process is arrested by the gastric fluid ; but the fluids from the
liver and pancreas renew the arrested operation when such matters have
been passed into the intestines. The albuminous compounds are in
considerable part dissolved by the gastric fluids; those passing from the
stomach undissolved are further acted upon by the intestinal fluids. The
oleaginous compounds are broken up, but are otherwise unacted upon by the
gastric fluids. When subjected to the action of the bile and pancreatic
fluid, they are broken up into minute globules covered by albuminous
matter. The more solid and fibrous parts of the food but little altered by
these agencies are passed on to the rectum, and thence defecated together
with the remaining unab-sorbed materials. The blood-vessels ramifying
through the inner coats of the stomach at once begin to absorb all soluble
compounds—saccharine, albuminous, and inorganic—which can penetrate the
enclosing membranes of the vessels. These absorbed compounds are passed
together with the venous blood through the several elaborating and
secreting organs, there to be prepared for assimilation in the tissues.
The compounds resulting from muscular action and the breaking up of tissue
substance are in part absorbed by the lymphatics, but principally by the
blood-vessels. The compounds absorbed by the lymphatics are supposed to be
such as are not entirely excrementitious, but still further capable of
organisation and assimilation. The blood is purified from all used up or
unnecessary compounds during their passage through the various excretory
organs. Albuminous
compounds pass by the several names of flesh formers, or of proteine,
plastic, and azotised, i.e., compounds containing nitro gen. Before
absorption they are converted into a compound named albuminose, which in
the blood soon assumes the forms of albumen and fibrin; the blood then
circulating in the capillaries effuses through their enclosing cellular
membranes into the tissues its fluid and gaseous contents; whereupon the
albuminous compounds go to build up and replace such bodies as the fibrin
and albumen contained in the muscular fibre of flesh, the casein in milk,
the gelatin in the bones, ligaments, horns, hoofs, hair, wool, &c, and
chondrin in cartilage or gristle. The oleaginous compounds, or fat
formers, supply material for storing up fat in the system. The adipose or
fat cells are congregated in nearly every part of the system. In the case
of animals fattened beyond natural requirements these cells are deposited
in increased quantity in all the soft tissues, thus increasing the size
and plumpness of the different parts of the frame; but at the same time
their presence greatly interferes with the continuance of muscular vigour.
They are also termed heat producers, as by the oxidation of their carbon
and hydrogen in the lungs and tissues they serve to maintain the requisite
temperature of the blood. The amylaceous and saccharine bodies are termed
the respiratory or heat-producing compounds. Their carbon and hydrogen are
oxidised in the lungs and other parts, and carbonic acid and water
produced thereby, as in the case of the last-mentioned compounds. It is
supposed that their excess in the blood is capable of conversion through
exchange of atoms into fatty material. As before mentioned, the production
of heat ensues upon all movements whatever of animal bodies. The
oleaginous and amylaceous compounds alike are incapable of supplying
flesh-forming materials, the reason being that they do not contain
nitrogen. There is a
peculiar modification of stomach represented amongst the ruminant animals
of the farm, those, namely, which are commonly said to "chew the cud." The
stomach in their case is divided into four different compartments, viz.,
1st (in order form the gullet), the rumen, or "paunch;" 2d, the reticulum,
or honeycombed bag; 3d the omasum, or "manyplies;" and 4th, the abomasum,
or stomach proper. The gullet or oesophagus is continued to the third
stomach; but in passing over the openings into the rumen and reticulum, it
is slit in such a manner that a small amount of pressure opens its folds,
and affords a passage into those two divisions. In the act of feeding,
ruminant animals, it will be seen, swallow their natural food before it is
thoroughly masticated. This, in being swallowed, deposits its bulkier and
more imperfectly chewed portions into the rumen and reticulum, through the
pressure of such portions upon the two folded openings into the same
respectively, the larger pieces naturally entering the rumen. And there it
remains, and is acted upon by the saliva swallowed along with it, until
the animal ceases browsing. Meanwhile the reticulum has been receiving the
supply of the rumen. When the animal, in the next place, commences to
ruminate, the contents of the reticulum are ejected through the folds of
its communicating aperture into the gullet in the form of small pellets,
and by an inverted muscular action of the gullet these are conveyed to the
mouth, where they undergo a thorough leisurely mastication. Reduced by
this process to a soft pulpy mass, its second passage down the gullet-is
unaccompanied with the requisite amount of pressure to open the passage
into its former receptacles, and it passes easily and smoothly to the
omasum. In like manner almost all fluid, softish or gruelly food, passes
directly to it in the first instance. It may almost be said that the
gullet is continued through the omasum into the fourth stomach. The inner
coats of the omasum are arranged as a great number of closely-placed
folds, and any substance imperfectly reduced, or triturated, so to speak,
passing along the continuation of the gullet, becomes perfectly reduced,
having to pass between these folds or "manyplies" before entering the
abomasum. Here finally, where is secreted the gastric juice, does
digestion proper commence. The abomasum leads directly into the duodenum.
Here, inasmuch as poultry form in many
instances an important branch of the live-stock of the farm, it may be as
well briefly to advert to the characteristic modification of the digestive
portion of their alimentary canal. Midway between mouth and stomach is
situated the ingluvies or crop, a pouch formed by folds or a species of
dilatation of the gullet; and here the grains and similar hard food
swallowed entire are stored up in the first instance. The food is
gradually passed to the gizzard, where it is crushed and ground up between
the muscular sides of this organ, which are lined with horny membrane. Its
peculiar action is greatly aided by the presence of the sand and minute
stones which the fowl instinctively swallows. The "proventriculis," or
stomach proper, has to be passed through, however, before the food enters
the gizzard. In the former is secreted the gastric juice, whence it is
that the food is subjected to its influence before being triturated by the
gizzard's action. The gizzard opens into the duodenum.
With regard to the reproductive process in
animals, the principal organs for producing and developing the embryo in
the female are the ovaries, in which are developed germ cells or ova, and
the uterus or womb, where the ova are impregnated by the male animal, and
where the ova are afterwards developed and sustained by the blood of the
mother until they are ripe for parturition. There are two ovaries, one on
either side, and they are situated in the region of the loins. They
communicate with the uterus by the Fallopian tubes, which are certain very
narrow channels. The ova, produced at periodical intervals, pass through
these tubes to the uterus, and their presence in that organ is made
evident by the phenomenon of "heat" or "rut" in the female. If sexual
intercourse, and the impregnation of one or more ova, do not supervene,
the ova are soon discharged from the body. In the opposite event the ovum
is retained in the womb, and there sustained by union with the maternal
blood circulation through the successive stages of embryonic growth, up to
the stage when it can maintain its separate existence as a fully developed
animal of the species ; whereupon the union is broken and the young animal
expelled from the mother's body by a wonderful muscular action of the
uterus. Until able to provide for itself, the young animal is fed with the
mother's milk, elaborated from the blood by her mammary glands. The testes
in the male animal are the corresponding organs to the ovaries; they
secrete the seminal fluid, containing sperm cells,—spermatozoids,—which
being discharged during the sexual act, and coming into contact with the
ova, serves to fertilise the latter. By castration, or the removal of the
testes, the male animal is rendered incapable of performing his share of
the reproductive process, but at the same time he becomes more docile in
disposition, and he is more easily and economically fattened than he could
have been before the deprivation. The female when deprived of the ovaries
assumes the like characteristics. In the case of fowls the ova, whether
fertilised or not, are expelled from the body in a complete state in the
form of eggs. They contain within the shell a thick layer of nutritious
albuminous matter,—the "white," —which affords nourishment, when
impregnation has been effected, to the growing embryo during the period of
incubation. The physiological functions of correlation hardly fall within
the scope of such a work as the present.
Chapter VI.—Of Meteorology.
The atmosphere, or aerial rind enclosing our
globe, is composed of a mixture of oxygen and nitrogen, and a small
proportion therein of one or two other gases. Purified air consists of 4
volumes or 77 parts by weight of nitrogen, and 1 volume or 23 parts by
weight of oxygen. But common air also contains a considerable quantity of
watery vapour, and carbonic acid as well, the latter in the proportion of
about 4 volumes to 10,000 volumes of air; and 1 part of ammonia to 1
million parts of air. Nitric acid is also present, but in minuter quantity
than ammonia. The height to which it continues above sea-level is
uncertain, and variously estimated at from 45 miles upwards. At the
sea-level its average pressure upon all objects is at the rate of 15 lbs.
on every square inch, and it can support a column of mercury 30 inches
high in a tube in vacuo, whose only open end enters the mercury contained
in an open vessel. Such an arrangement constitutes the invaluable
instrument, the barometer, the measurer of atmospheric pressure, and as
such, an indefinite multiplier of science. Aerial density gradually
diminishes as we ascend above the sea-level, and with it aerial pressure
as well. Heat expands air, which decreasing in density, ascends; its
vacant place being occupied by a fresh supply possessed of the normal
coldness and density. Hence the origin of the winds and the constant
atmospheric movements round the earth. The constant ascent in this manner
of the body of air superincumbent upon the tracts of ocean, having a
breadth of belt extending to one or two degrees on either side the
equator, and the supply of colder air to fill up the vacuum, combine to
effect the beneficial phenomenon of the steady trade winds. And their
prevailing direction is consequent upon a supplementary fact, viz the
greater speed of terrestrial revolution at the equator, arising from the
earth's increased diameter at that part; for this swifter easterly motion
than at other parts of the earth's surface makes the trade winds fall
behind, so to speak, and to seem blowing from the north-east and
south-east. To the irregular occurrence of partial aerial rarefaction is
due the phenomena of monsoons and all winds great and small. From water,
moisture evaporates at all temperatures, even when it is in the form of
ice or snow and the air has the property of being able to hold a large
quantity of such aqueous vapour in suspension. The higher the temperature
of the atmosphere, the larger the proportion of aqueous vapour it can
absorb; therefore it possesses various degrees of saturation, i.e., points
beyond which it can hold no more in suspense. As air becomes cooler, so
does its power of saturation also become lowered; whereupon all the watery
vapour in excess is condensed, and falls as rain, or is deposited as dew.
Dew is produced by the rapid radiation of heat from the warm
surface-ground and herbage after sunset These becoming cooler than the
immediately surrounding air, the latter in turn parts with its heat to
them by radiation, whereby it has its saturation point lowered, and the
excess of vapour becomes deposited as dew. And so with fogs and mists;
they result from the radiation of heat from land and water, taking with it
aqueous vapour, which becomes visible upon encountering cooler air.
Similarly rain is produced when heated volumes of air are deprived of
their heat, through the fall of condensed vapour, which assumes, according
to the temperature it encounters, the form of ram, hail, or snow. The
following table gives the weight in grains of a cubic foot of vapour at
successive ascents of 10° from 0° to 90° Fahrenheit, clearly demonstrating
the increase of the saturation point with the rise of temperature:-
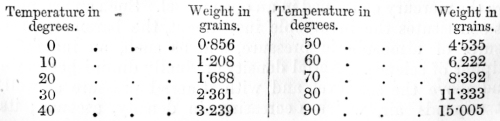
"When by any cause the temperature of the air
is reduced, its particles (molecules), approach nearer each other, and so
do those of the vapours held suspended in the air; and as steam becomes
visible when mixed with atmospheric air, so vapour becomes visible when it
suffers condensation by a reduction of temperature, and then becomes
clouds. These differ much in altitude and size." In this way we can
visibly perceive the contained watery vapour in our lung exhalations
during frosty weather, as also at such times as the atmosphere is already
saturated with moisture.
On this natural provision of the aerial
absorption of moisture depends the entire system of circulation of water
from earth to sea, and vice versa. From the immense tracts of the tropical
seas, and those of less heated zones, enormous volumes of water are being
continually uplifted into the thirsty air. And this saturated air,
becoming impelled in every direction by all the winds that blow, meets
with cold elevations and land surfaces, or colder currents; whereby,
losing its excess of temperature by means of radiation, its corresponding
excess of moisture is condensed, and descends as rain to promote vegetable
life, to fill our springs, rivers, and lakes, and take a leading part in
effecting the physical and chemical changes which are constantly
occurring, and altering the general contour of the world.
The frequency rather than the amount of
rainfall, indicates the atmospheric humidity of any district. Generally
speaking, the number of days in a year on which there falls rain,
increases as we recede from the equator to the temperate zones ; and the
amount of rainfall decreases with distance from the equator with increased
elevation above sea-level, and with distance from the sea:—
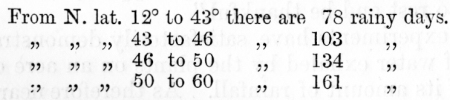
The rainfall of the tropics is estimated at
95, and of the temperate zones at 34 inches annually. In some parts of the
West Indies, as much as 600 inches have fallen in one year.
In the comparatively small area of Great
Britain there is yet large diversity of the amount of rainfall, humidity,
and temperature of the atmosphere. This, besides being due to local
physical causes, arises from others prevailing over a large portion of the
earth.
In some districts sheltered by higher ground
from unfavourable winds, the temperature is much higher than it is in less
favoured districts, and from the condensing action of the hills the
humidity is also greater. The prevailing westerly winds come
moisture-laden from the Atlantic into contact with the colder surface of
the western parts of our islands; they become lowered in temperature, and
consequently part with much of their moisture at the place of contact, in
the form of mists and rain; and thus they keep these parts at once warmer
and more humid than the eastern districts of the kingdom. Such influences
have, by necessity, a considerable bearing upon the question as to the
preferable system of agriculture for adoption in these districts
respectively. For whilst the possession of an atmosphere humid to excess,
with its attendant want of frequent sunshine, renders certain districts
incapable of properly maturing our more valuable cereal crops, they are at
the same time better fitted for the cultivation of the important green
crops, and thereby better calculated for the successful practice of the
several systems of stock-farming than other, in some respects, more highly
favoured counties.
The following remarks of the English
Registrar-General are of interest in this connection. He says:—"Rain fell
in London to the amount of 43 inches, which is equivalent to 4300 tons of
rain per acre. The rainfall during last week" (February 1865) "varied from
30 tons per acre in Edinburgh, to 215 tons per acre in Glasgow. An English
acre consists of 6,272,640 square inches, and an inch deep of rain on an
acre yields 6,272,640 cubic inches of water, which at 277,274 cubic inches
to the gallon makes 22,622.5 gallons; and as a gallon of distilled water
weighs 10 lbs., the rainfall on an acre is 226,225 lbs. avoirdupois; but
2240 lbs. are a ton, and consequently an inch deep of rain weighs 100.993
tons, or nearly 101 tons per acre. For every 100th of an inch, a ton of
water falls per acre. If any agriculturist were to try the experiment of
distributing artificially that which nature so bountifully supplies, he
would soon feel inclined to rest and be thankful."
Numerous experiments have satisfactorily
demonstrated that the amount of water exhaled by the plants on an acre of
ground is in excess of its amount of rainfall. As therefore nearly 2-5ths
of the total rainfall are carried away by the drainage, it will be better
judged to what an extent takes place an almost insensible circulation of
water from earth to atmosphere and reversely.
The atmospheric temperature decreases as we
approach the higher latitudes from the equator, and also with increased
elevation above the sea-level. For every 300 feet of ascent above the
sea-level the mean or average temperature decreases 1°. But with
increasing distance from the equator there is no uniform gradient of
decrease, owing to the unequal distribution of sea and land and other
causes producing a variation of temperature in parts of the world included
in the same degrees of latitude. The lines which cover tracks of the world
having the same mean temperature are called "isothermal lines." The
atmospheric temperature is measured and indicated by the thermometer,
which contains mercury or fluid in a closed glass tube, from which the
atmospheric air has been extracted. Its contents expand and contract under
the influence of heat and cold; and the amount of these respectively is
indicated by the standard scale of degrees according to which the
instrument is graduated. They are also constructed with an arrangement for
registering the maximum amount of cold which has been reached during any
fixed period. The hygrometer indicates the amount of moisture contained in
the atmosphere immediately surrounding it; whilst the hygro-scope again
merely indicates its presence. Anemometers are instruments used for
measuring the amount of the wind's force and velocity. As exhibiting the
influence of temperature upon the distribution of plant life in the earth,
we find the face of the globe in physical atlases, from the equator to the
pole, roughly divided into eight isothermal zones, with characteristic
plants, as follows:—
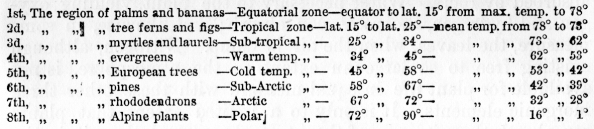
The same applies to the vertical isothermal
lines, i.e., those indicative of similarity of temperature in distance
above sea-level. We can see the effects of temperature on the choice of
cultivated plants exemplified within the limited area of our own country,
in the presence and absence of certain field crops, as we travel from the
southern to the northern extremity of our island. The important bearing
which the science of meteorology brings more immediately upon agricultural
practice has until recently been almost entirely overlooked; but now its
principles, and their connection with the flourishing of farm crops and
the welfare of live-stock, are more entirely appreciated. And there is all
the more reason for this, from the peculiar situation of our kingdom
subjecting it to numerous, extreme, and sudden changes of weather. The
farmer can now, at the same time, more successfully combat with these,
through the agency of the widely-disseminated weather-charts and
forecasts, the fruits of the system so admirably organised by the
Government department of the Board of Trade.
Chapter VII.
Of the Leading Scientific Principles of the
Art of Agriculture.
The perusal of the foregoing chapters will
have prepared the student for tracing the relation of the various physical
sciences therein successively treated of, to the leading principles of
agriculture, as they will next be practically adverted to in the remaining
portion of this manual.
The classification and the chemical and
physical characteristics of soils will fall to be considered by way of
preliminary matter, seeing that the soil is the primary essential
requisite for affording the plant a foundation on which to begin the
formation of its structure, and, at the same time, in connection with the
atmosphere, supplies all the elements necessary for the plant's growth.
And before doing so a brief recapitulation is expedient of the plant's
mode of absorbing its organic and inorganic constituents. The plant is but
little dependent on the soil as a source of carbonic acid supply, as it is
the carbon of the atmosphere which it is constantly absorbing and
assimilating. Still, a considerable quantity of the atmospheric carbon is
being carried into the soil when the former is dissolved by rain water.
And when it is there freed by chemical action a proportion of it is
directly absorbed by roots. Water necessary to the plant, yielding oxygen
and hydrogen, the roots can absorb; and likewise, in some measure, the
leaves, when the air is damp. Nitrogen, although existing free to so large
an extent in the atmosphere, is not available for plant life in equal
measure with these other three inorganic elements. It is only to a limited
extent that plants can absorb the nitrogen of the atmosphere; and then it
is the -ammonia and nitric acid it contains and only from the ground as a
source, into which, both being soluble in water, they are carried by the
rain. On this point Liebig says:—"As regards the quantity of ammonia thus
brought down by the rain, ... as 1132 cubic feet of air, saturated with
aqueous vapour, at 59° Fahrenheit, should yield 1 lb. of rain water, if
the pound contain only l-4th of a grain of ammonia, a piece of ground of
26,910 square feet—43,560 square feet being an acre—must receive annually
upwards of 80 lbs. of ammonia, or 65 lbs. of nitrogen; which is much more
nitrogen than is contained in the form of vegetable albumen and gluten in
2650 lbs. of wood, 2500 lbs. of hay, or 200 cwt. of beetroot, which are
the yearly produce of such a piece of ground ; but it is less than the
straw, roots, and grain of corn which might grow on the same surface would
contain." Other chemists, however, calculate the amount of nitrogen
carried by rain to the soil at a much lower figure than the Baron does.
Humus, or the organic matter of soils, absorbs ammonia from the
atmosphere, and when existing free in the soil; in like manner does clay.
Nitric acid and ammonia are also produced in the soil by the decay of
animal and vegetable substances containing nitrogen. The former combines
with bases, such as potash, soda, or lime, to form nitrates; and these and
the ammonia become absorbed by the clay or humus. It is understood that
plants absorb the liberated ammonia in a direct manner, and nitric acid in
the same way; although in regard to the latter the question is more
undecided. These, accordingly, are the natural sources whence plant life
obtains the necessary organic elements of carbon, hydrogen, oxygen, and
nitrogen. The inorganic compounds, whence are derived the inorganic
elements necessary for the upbuilding of plant structure, exist in the
soil in, as we have seen, an almost insoluble condition. But rain water
holding carbonic acid in solution has, we know, its soluble power over
such compounds in the soil vastly increased. But even with this, and the
additional fact of so large a quantity of it permeating the soil and plant
structure (experiment has shown that for every grain of inorganic matter
assimilated by a plant, 2000 grains of water have passed through the
latter), still plant life could not derive inorganic substance in
sufficient quantity, without the additional agency of the power stated to
be inherent in roots, of contributing by chemical or other means to the
solubility of the compounds of this class with which they come into
contact in the soil. By heat and light these several absorbed compounds,
when exposed in the leaf or elsewhere, are broken up, and their atoms and
molecules rearranged, to form the proximate and other compounds found in
plants.
The several constituents of plant life "all form," says Johnston, "more or
less constantly and abundantly a portion of the fixed and solid matter of
the plant taken as a whole. They may not be found in any one part of the
plant when separated carefully from the rest; but in the solid parts of
the plant, taken as a whole, they are all and always to be met with. When
thus deposited they become, for the most part, dormant, as it were; and
for the time cease to perform an active chemical function in the general
growth; though, as vessels or cells, they may still perform a mechanical
function. They undergo various chemical changes in the interior, chiefly
while circulating or contained in the sap, by which changes they are
prepared and fitted for entering, when and where it is necessary, into the
composition of the solid or fixed parts of the plants. Thus the starch of
the seed is changed into the soluble dextrin and sugar of the young plant,
and then again into the insoluble cellular fibre of the stem or wood as
the plant grows ; and finally, into the insoluble starch of the grain as
its seed fills and ripens. They each exercise a chemical action more or
less distinct, decided, and intelligible upon the other elementary bodies,
and the compounds of them, which they meet with in the sap of the plant.
In regard to some substances, such as potash and soda, the sulphuric and
phosphoric acids, this last function appears to be especially important.;
These substances influence all the chemical changes which go on in the
interior of the plant, and which modify or cause its growth.
The same is true of the nitrogen which the
plant contains. This elementary body, in the form of albumen, or some
other of the numerous protein compounds which occur in the sap, presides
over, or takes part in, almost every important transformation which the
organic matter of the living vegetable undergoes. Thus it is always
abundantly present when the starch of the seed or of the tuber is
dissolved and sent up to feed the young shoot; and again, when the soluble
substances of the sap are converted into the starch of the grain of the
tuber, or of the body or pith of the tree, one or other of the protein
combinations is always found to be present on the spot where the chemical
change or transformation is going on. Besides these general functions, the
several substances found in plants exercise also special functions in
reference to vegetable life and growth. Thus, nitrogen is most abundant in
the sap of young plants, takes part in most of the changes of organic
compounds which go on in the sap, and fixes itself, as the plant
approaches maturity, in greatest abundance in the seeds and in the green
leaves. Nitrogenous manure alone produces negative results. Potash and
soda circulate in the sap, influence chemical changes very much, and
reside or fix themselves most abundantly in green and fleshy leaves, and
in bulbous roots. Sulphuric acid is very influential in all chemical
changes, is found in most cases in those parts of the plant in which soda
and potash abound, and deposits a portion of its sulphur wherever the
compounds of nitrogen form a notable part of the substance of the plant.
Phosphoric acid exercises also much influence over the chemical changes of
the sap, and finally fixes itself in greatest abundance in the seeds and
other reproductive parts of the plant. Soluble phosphates, from whatever
source derived, produce no difference, whether as dissolved guano,
coprolites, or bone ash. Lime is very important to healthy vegetable
growth, as practical experience has long testified. Among other duties, it
appears to accompany the phosphoric acid in the sap of plants, and to
deposit itself in combination with organic acids in the leaves and bark,
and with phosphoric acid in some seeds and roots. Magnesia appears also to
attach itself very much to phosphoric acid in the sap, and fixes itself in
combination with the acid principally in the seed. Chlorine—the chemical
function of this substance in the sap is less understood even than that of
the other substances above-mentioned. It exists chiefly in combination
with soda, and is much more abundantly present in some plants, and in some
parts of plants, than in others. Though, as I have said, its immediate
chemical function in the plant is not understood, it forms a most
important constituent of the plant, in so far as the after uses of
vegetables in the feeding of animals are concerned. Silica exists in the
sap in a soluble form, and deposits itself chiefly in the exterior
portions of the stems and leaves of plants. It is supposed there to serve
as a defence to the plant against external injury, and to give strength to
the stem in the case of the grasses and corn-yielding plants; but what
chemical functions it performs, if any, in directly promoting vegetable
growth, we can scarcely as yet even venture to guess."
The following analysis of a good arable light
sandy loam by Anderson will give an idea of the manner in which the
several elements are combined in the soil, although the several salts are
broken up into their component acids and bases:—
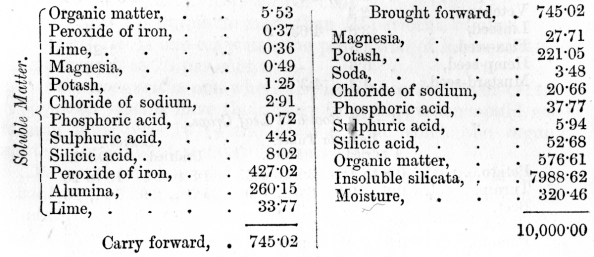
Soils.
From the difficulties which attend the
obtaining of complete analyses of soils, no large amount of attention has
hitherto been devoted to this branch of agricultural chemistry, except in
instances where the object has been to determine the quality of some of
the more important and indispensable constituents, such as lime. It will
easily be granted, what a variety the analyses of different soils must
present. The width of variation must he obvious, which exists between the
composition of the soil of a chalky district and of soil taken from a
reclaimed peatmoss. And again, whilst the analyses of two soils may show
an almost identical composition, their measure of fertility may still be
very unequal, owing probably to the soluble nature of the components of
the one, and the different conditions of combination, and lessened degree
of solubility prevailing with the constituents of the other.
In connection with the analysis last above
given, the following table, extracted from Stephens's "Book of the Farm,"
give the amount of ash or inorganic material taken from the soil by some
of our cultivated plants—the quantity being for every 100 lbs. of each
plant:—
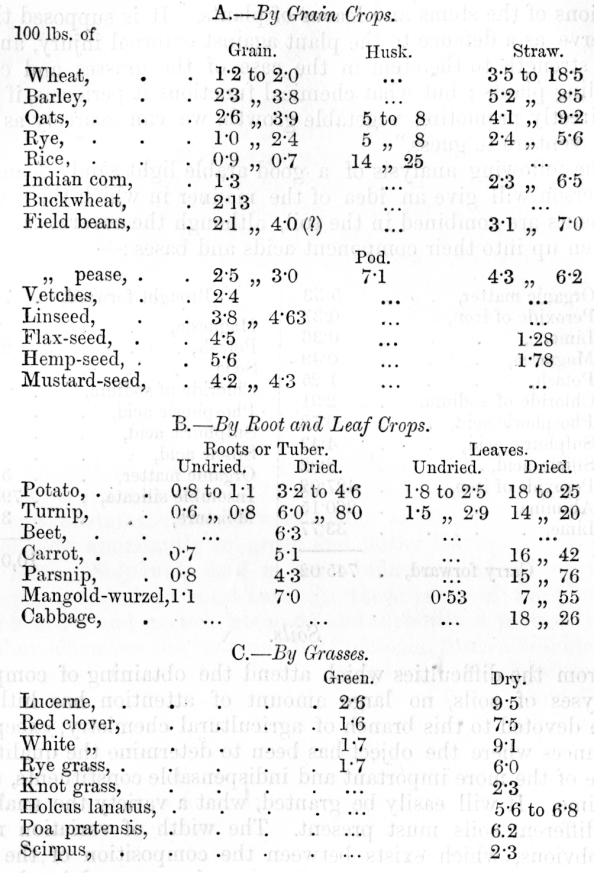
From these tables we perceive what a small
percentage of the weight of plants is contributed by the inorganic
materials of the soil.
Clay and sand are the two substances which
determine the texture of the soil; and to the variety of their admixture,
in regard to their respective proportions, are due the different classes
of soils. The following classification of soils, as proposed by Johnston,
may be selected as being the simplest, and that one, at the same time, by
whose standard the classification of any particular soil may most easily
be determined.
The purest clay, such as pipe-clay, found
naturally, consists of silica, alumina, and a small quantity of oxide of
iron, in chemical combination. No sand can be extracted from it. Tile
clay, the strongest of soils, consists of pure clay, mixed with from 5 to
15 per cent. of siliceous sand; this can be separated from the clay by
boiling, or otherwise thoroughly incorporating the clay with water, and
then allowing the mixture to settle. The sand settles first, and the
liquid can be poured off just as the finely divided clay begins to be
precipitated at the bottom of the vessel.
A clay loam permits of from 15 to 30
per cent. of fine sand to be separated from it in this manner.
A loam, from 30 to 60 per cent.
A sandy loam, from 60 to 90 per cent.
A sandy soil contains no more than 10 per cent. of clay.
A marly soil is one on which the proportion of lime contained
ranges from 5 to 20 per cent.
A calcareous soil is one where the lime exceeds 20 per cent.
Vegetable moulds have their range from the garden mould, containing
from 5 to 10 to the peaty soil in which the organic matter may amount to
60 or 70 per cent.
The last three classes of soils are also
clayey, loamy, or sandy, according to the description of the predominant
element of the admixture. This organic matter or humus springs, as we have
seen, from vegetable decay, When abundant, as in the case of peaty soils,
it is inactive, and inorganic matter is deficient in quantity. But after
drainage, and in regular cultivation, humus rapidly decays, and gets used
up till the subsoil is reached and incorporated with the remaining humus,
when there is found a more normal soil.
The amount of humus persistent in our
cultivated soils is rarely less than 5 per cent. Chemically, it is a
mixture of several acids, varied according to its stages of decay, all
composed of carbon, hydrogen, and oxygen. It is not considered to be a
direct source of food to the plant, but it is of great importance as a
chemical agent for effecting changes in the soil. As previously mentioned,
it absorbs ammonia from the atmosphere, and when free in the soil, and
also soluble alkaline compounds, thereby preventing their being washed out
of the soil; and at the same time so retaining them, that they can easily
be liberated and absorbed by the plant roots. It likewise absorbs oxygen
from the atmosphere, and freeing it in the soil, maintains a constant
chemical action amongst the several constituents. Clay possesses similar
absorbent properties, and its presence is therefore of considerable value,
when not so intrusive as to impede the exercise of proper cultivation.
With regard to the matters carried away in
solution by that portion of the rainfall which is drained out of the soil,
Prof. Anderson, in commenting on the analyses of different drainage water
from various soils—the rainfall estimated at 25 inches—by Way and Krocker,
says:—"It appears that about 2/5 of all the rain which falls escapes
through the drains, and the rest is got rid of by evaporation. An inch of
rain falling on an imperial acre, weighs rather more than a hundred tons;
hence, in the course of a year, there must pass off by the drains about
1000 tons of drainage water, carrying with it, out of the reach of the
plants, such substances as it has dissolved, and 1500 tons must remain to
give to the plant all that it holds in solution." (It has been already
stated, however, that the amount of moisture inhaled by the plants
covering an acre of ground alone exceeds the rainfall on such an area.)
These 1500 tons of water must, if they have the same composition as that
which escapes, contain only 2½ lbs. of potash and less than 1 lb, of
ammonia. It may be alleged that the water which remains, lying longer in
contact with the soil, may contain a large quantity of matter in solution,
but even admitting this to be the case, it cannot for a moment be supposed
that they can ever amount to more than a very small fraction of what is
required for a single crop. It may, therefore, be stated with certainty
that solubility in water is not essential to the absorption of substances
by the plant, which must possess the power of itself directly attacking,
acting chemically on, and dissolving them. The mode in which it does this
is entirely unknown, but it, in all probability, depends upon very feeble
chemical action, and hence the importance of having the soil constituents,
not in solution, but in such a state that they may be readily made soluble
by the plants," viz., with the particles in a finely divided state. The
nitrates being the most soluble salts are washed out most abundantly.
We speak of soils as being stiff, tenacious
and heavy, when they are difficult to cultivate by means of the ordinary
farm power; sharp and free when they are of a gritty texture, and easily
pulverised; deaf, when of a spongy, inactive texture, as when the soil
adheres to the iron of the plough and other implements; deep, when they
can be deeply furrowed and stirred without the subsoil being reached;
thin, in the opposite case; retentive, when they retain the surface water,
and admit only of its slow percolation downwards; and porous when the
reverse takes place. Such other terms are applied, as rich, poor, hungry,
grateful, kindly, and so on, according to the degree of natural fertility
in soils, the readiness with which they absorb and retain manurial matter
added to them; or, on the other hand, with which they part with it unused,
or their possession, or want of other such like obvious properties.
Black soils are to be seen in peaty, white
soils in chalky districts. Red soils are frequent, and for the most part
they are very fertile. But the prevalent tint is a brownish one. Black,
dark-coloured soils reflect the solar rays less, and consequently absorb
more of their heat than do the whitish, light-coloured sorts ; but from
their property of radiation, they part with heat more quickly than do the
latter. Soils, exposed to the sun's hottest rays at right angles, absorb
more heat than if otherwise situated. Clay soils, and such as have an
excess of humus, absorb more moisture and keep it longer than do sandy
soils, and such as contain a less proportion of organic matter.
Drainage.—To the end that cultivated plants
may flourish, it is absolutely necessary that the soil be relieved from
the presence of stagnant water; for the presence of such water, besides
continually lowering the temperature of the soil, by the constant demands
it makes upon the soil's heat in the act of evaporation, also precludes
the possibility of air circulation through the soil's component particles:
The first step, accordingly, in good husbandry, when the soil is not
naturally dryish, is its artificial drainage ; which process having been
effected, and the rain water now percolating through the soil into the
drains, and only so much of it as is retained in the soil by capillary
attraction becoming evaporable, the soil being thereby deprived of less
heat, has its temperature raised considerably, promotes the earlier
germination of seeds, and brings all the crops grown upon it to a speedier
maturity. Land is also thus rendered capable of being worked at times and
seasons in which it was formerly unworkable, and always with a less
expenditure of labour. The common ways of constructing the underground
ducts of drainage works in agriculture are sufficiently familiar. Clay
pipes of varying shape and bore, and laid continuously in parallel rows,
are usually employed, though in certain districts recourse is had to flat
stones, so arranged as to form a channel with triangular-shaped bore. The
surplus water then percolates the soil, and meeting the drains, leaks or
soaks through the joints imperfectly fitting of the clay or stone
conduits, and by gravitation it is carried through these to some clear
outlet.
The Rotation of Crops.
In like manner, as the strength of any
mechanical chain is regulated by its weakest link, so may the fertility of
any given soil be said to depend (other conditions being favourable) on
that one essential element or compound which is present in it in the least
quantity of all. To the recognition of this, and the additional
ascertained fact, that some plants require a greater amount of particular
elements or compounds than others do, is due the custom, in agricultural
practice, of observing a "rotation" or varied succession of crops, whereby
it is possible to obtain permanently a maintenance of the proper balance
of the soil's constituents. The annexed table, composed by Baron Liebig,
in which the whole inorganic materials assimilated by plants are included
under the three heads of salts of potash and soda, of lime and magnesia,
and of silica, bears out the fact:—
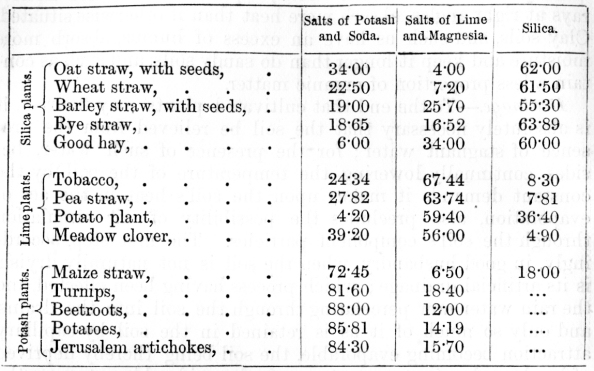
Until quite recently, the general opinion was
entertained that by the continuous growing of one particular species of
crop on the same piece of ground, and that even when the appropriate
manures were supplied to it, both quantity and quality of the returns
gradually diminished and deteriorated, until at length its cultivation
proved fruitless. As accounting for this, the theory was advanced of
plants excreting matter from their roots, and that any accumulation of
this was hurtful to plants of the same species as the excreting one. But
this opinion must now be surrendered; and where failures occurred in
cultivation after such a sort, they were due, doubtless, to mistaken ideas
as to the requisite manures, and the neglect of adopting means for the
check of fungoid and insect ravages. Ample proof is now afforded by
results in the case of grain crops, that the same species of crop may be
continuously raised on the same plot of ground with profit, provided
suitable manures are applied, freedom from weeds secured, and the ravages
of insects and fungi averted. But hitherto this admission has had but
little effect in altering the customary and fixed rotations of cropping
peculiar to different districts and estates. No doubt, so long as the
great majority of soils are subjected to the present mixed method of
husbandry, which must of necessity accompany the rearing and feeding of
live-stock, rotation of cropping must continue as an institution,
providing as it does for the requisite proportions of litter and dry and
green food for the various species of animals. Its different systems are
determined by the nature of climate and soil, the situation and demand of
the more convenient markets, and the like considerations. But previously
to observing in detail some of the ordinary rotations, it will here be
expedient to notice succinctly the leading characteristics of our
principal cultivated plants, the soils to which they are naturally best
adapted, and the elements which they demand in greatest quantity from the
soil. Wheat is the most important of our cereal crops, as it possesses the
proximate constituents in the proportion best suited for man's nutrition
in temperate climates ; it is therefore the staple bread-corn of the
northern temperate zone. Its range of cultivation is wide, extending
between the tropics and the isothermal line denoting the descent of the
mean annual temperature to 56°. Also, it is cultivated in a great variety
of soils ; clayey loams suit it best; and its returns are more profitable
from the stronger than a light class of soils. The average composition of
the grain is indicated by the figures following:—
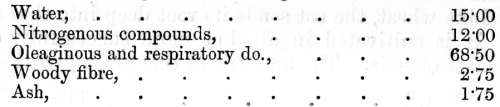
And the average of several analyses of the ash
or mineral matter gives the following results:—
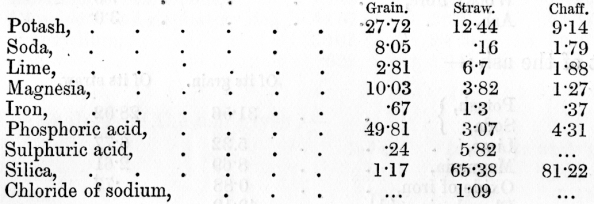
Barley has even a wider range of cultivation
than wheat, maturing in almost every climate in the world. Unlike the
wheat plant, whose roots penetrate deeply in the soil and subsoil, those
of barley ramify in the looser material nearer the surface, sending few
down into the subsoil, but developing increased numbers of fibrils and
hairs, so that its absorbent powers are very great.
It matures much quicker than the other grain
plants; a period of from seven to eight weeks between seed time and
harvest often suffices. It is best suited with light free soils. The
analysis of the grain is as follows:—
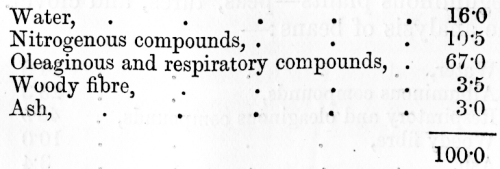
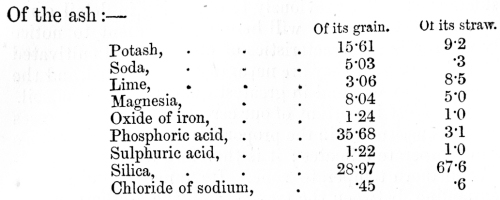
Oats are also cultivated throughout a wide
range, but they are better suited for countries possessing a lower annual
temperature, less sunshine, and a moister climate than those in which the
cultivation of wheat and barley is extensively followed. In Scotland, the
breadth under oats considerably exceeds that under wheat or barley. Like
wheat, the oat sends its root deep into the soil and subsoil. It is
cultivated in all, but more successfully on the stronger class of soils.
The analysis of this grain gives:—
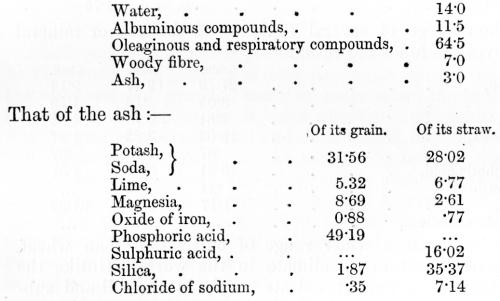
The Bean plant is grown with most profit on
strong soils; but it is not cultivated to anything approaching the same
extent as the cereals last mentioned, as it enters but little into the
composition of our bread stuffs. It is chiefly used for stock-feeding
purposes, and from the large proportion of albuminous compounds which it
contains, it is especially valuable in the maintenance of muscle in hard
working animals. This also applies to the other cultivated leguminous
plants—peas, tares, and clover. The following is the analysis of beans:—
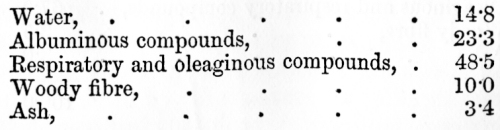
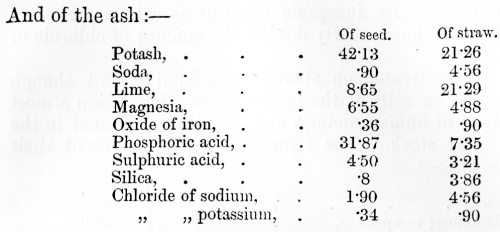
The Turnip is a plant essentially suited to
deep, loamy, light, and free soils, for these easily admit of thorough
pulverisation, a condition of soil necessary for the development of the
bulb, and the descent of its tap root, with its numerous diverging
fibrils. As can easily be understood, from the numerous cultivated
varieties, and the variety of soils in which turnips are sown, great
diversity appears on analysis, but the following may be taken as
averages:—
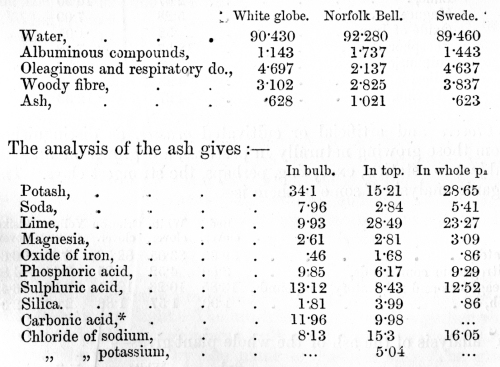
* Formed by the oxidation of the carbon.
The Mangold-wurzel, unlike the turnip,
succeeds well in the strongest clay soils, though it is best suited for
the medium class of these. On the European continent it is extensively
cultivated for the manufacture from its saccharine compounds of the common
sugar of commerce. It is largely substituted for turnips in the English
midlands and eastern and southern counties, and in Ireland also. The
organic analysis of the mangold is very similar to that of the turnip, but
showing a larger percentage of non-nitrogenous bodies; its inorganic
analysis is similar as well, excepting that it shows nearly double the
amount of chloride of sodium.
Potatoes are cultivated on almost every kind
of soil, though they thrive best on soils of the lighter class. They form
a most important item of human dietary, and are also much used in the
feeding of farm stock. The figures following represent their average
analysis:—
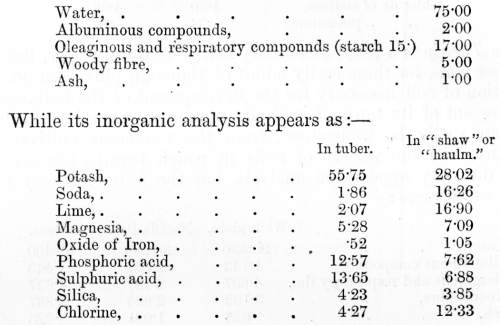
Clovers and artificial or cultivated grasses,
as distinguished from those growing naturally in pastures, are grown on
all soils subject to rotation, excepting, perhaps, the strongest clays.
The organic analysis of some of them is—
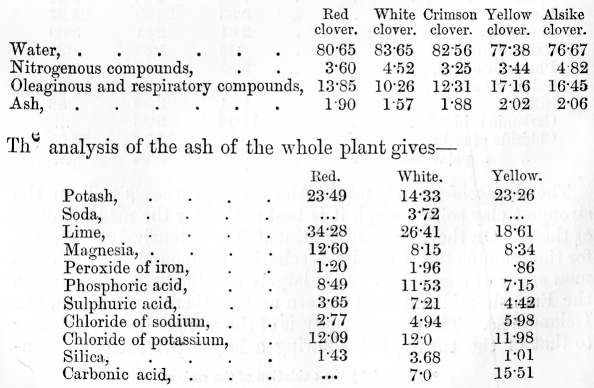
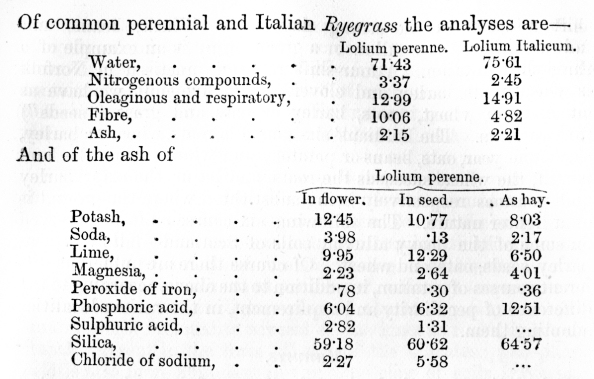
By conversion into hay, grasses and clovers
lose about three-fourths of their weight of water.
Whatever may be considered the necessity of
rotations as a preserver of the due balance of the soil's constituents,
they at all events afford facilities for the periodical cleansing of the
ground from the weeds, which at a subsequent period of any rotation might
flourish in a particular crop, the nature of which might preclude any such
purification ; as in the case of grain crops, for instance, sown broadcast
or too closely drilled to admit of the intervening soil being hoed. The
crops in a rotation which allow of a thorough cleansing from weeds being
effected are turnips and the like, mangolds, potatoes, and so on. As these
several crops are drilled at a sufficient distance apart to permit the
passage of horse hoes between the rows, the soil can be thoroughly kept
free from weeds till the possibility of their flourishing is taken away by
the growth of the crops to such a point as to effectually protect and
shade the intermediate spaces from free air and light. The crops
last-mentioned, together with grasses and other forage plants cultivated
for the sake of their roots or leaves, pass by the name of green crops.
The grain crops, on the other hand, or those cultivated for their seeds,
are called white crops, com crops, or cereals.
Formerly it was a prevalent custom at stated
intervals to leave portions of ground uncropped for a whole season,
repeated ploughing and other workings being granted to it during the
summer, in order to destroy the weeds as far as possible. This was called
giving the land a bare or naked fallow. This practice is still had on
strong soils, but it is gradually yielding to that of taking out of the
soil some green fallowing crops, e.g., cabbage, which is well suited to
strong soils. Wheat and beans in succession, with an occasional bare
fallow is an example of a two shift rotation on the strong clay soils.
Wheat, beans, and fallow (either a bare fallow or a green crop) is an
example of a three-shift rotation. A four-shift rotation practised in
Norfolk is wheat, roots, barley, and clover. Of a five-shift, we have as
an example, wheat, roots, barley or oats, and grass ("seeds") for two
years. The Lothians' six-course is roots, wheat or barley, seeds one year,
oats, beans or potatoes, and wheat; when the land is stiff, the wheat
succeeds the roots, and beans the oats; barley and potatoes respectively
being substituted where the ground is of a lighter nature. The following
six-course shift is observed on some of the heavy alluvial soils of
Scotland—fallow, wheat, barley, seeds, oats, and wheat. Of course there
are numerous different courses of rotation, in addition to the above,
according to the difference of peculiarity and requirement, in the various
localities adopting them.
Manures.
Calculating upon the basis of the figures of
the analyses, quoted in the preceding chapter, an average crop of wheat
extracts, per acre, from the soil, inorganic compounds to the following
amount:—
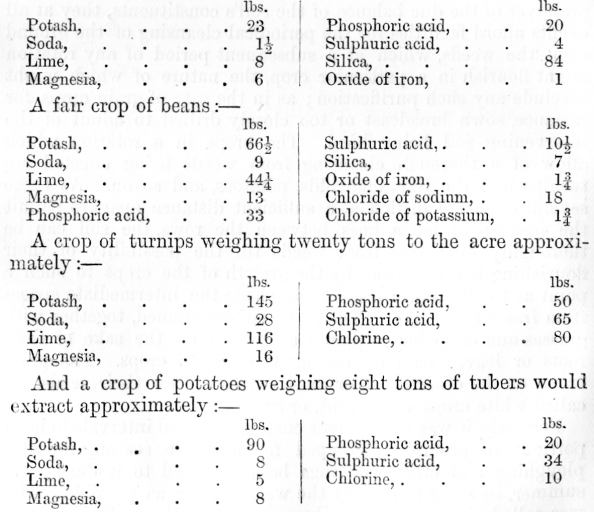
carried away from the soil in quantity
proportionate to the analysis of the plant. Cows remove more inorganic
matter from the soil than fattening animals, from the fact of their milk
containing a considerable amount of such materials, not again to be
delivered to the soil. In like manner, young cattle, from their requiring
frame-building constituents, remove more than does fattening stock.
Phosphoric acid, then, and potash, especially
the former, are the most valuable of the soil's inorganic compounds, by
reason of their being the scarcest among them, but at the same time
requisite in considerable quantity for the health of cultivated plants.
The quantity of the remaining constituents is sufficiently contained by
most soils for ordinary requirements. Potash is scarcest in light soils;
in the strong clay soils and sundry closely related to several of the
igneous rocks, it is constantly present in the form of one of the
silicates; phosphoric acid is also more abundant in the same class of
soils. We saw, that of the organic elements, that one least available to
plant life was nitrogen; for all which reasons it is, that ammonia or
nitric acid, and phosphoric acid form the bases of all manufactured
manures ; which are called nitrogenous or phosphatic, according to the
nature of the basis prevailing. Before it was commenced to manufacture
mixtures of the necessary constituents, they were principally derived for
return to the soil from the natural sources of farm-yard manure and town
sweepings and refuse. The farm-yard manure was applied to so much of the
ground as it could be spread over, the remaining arable or cultivated land
was bare fallowed for a season; and, indeed, a bare fallow, in certain
respects, resembles a manuring, for besides affording the opportunity of
thoroughly cleansing the land from weeds, the frequent ploughings and
dressings it undergoes expose its whole substance to the atmospheric
action, which renders such important chemical changes, and breaks up
insoluble compounds, fitting them for plant consumption in the next
season.
When the situation afforded facilities for the
purpose, town manure and other waste were turned to account. But a demand
for other manures than these sprang up with the introduction of turnip
cultivation and generally improved farming. Guano was imported, recourse
was had to the use of bones; and at length the way was opened up to the
production of artificial manures suitable for all kinds of crops. And now
the market is full of manures compounded for the specific requirements of
all our cultivated varieties, and all manner of waste and all refuse, in
any respects qualified to return plant-food to the soil, are well
economised, with the exception of town sewage, perhaps; for the many
difficulties preventing its profitable application to the soil yet remain
to be overcome. When we consider the fact of our country not producing of
itself sufficient food for the population, the importation of the extra
quantity needed from abroad, and the immense amount of manure stuffs now
applied, and when, in addition we bring to mind the truths of all chemical
elements being indestructible, and all such as compose the food of man and
the lower animals being voided, some as gases, indeed, but the vast
proportion in the form of fluids and solids, it must be allowed, that if
its soil were the recipient of all these which are now lost, the fertility
of Great Britain would naturally increase at an indefinite ratio. But, on
the contrary, we see a gigantic illustration of wastefulness—the excreta
of the inhabitants, and the other valuable manurial substances of our
great cities and towns, poured into rivers, which are converted by the
process into gigantic open sewers fatal to life, rolling everything to the
sea. Where a regular course of rotation is observed, the general rule is,
to apply to the green crops all the farm-yard, manure with others, in
quantity sufficient for all the requirements of every subsequent crop of
the rotation. But the practice of supplementing this application by
another of specific manures for the successive crops, is now rapidly
extending, under the influence of scientific farming.
Farm-yard manure, consisting as it does of the
excreta of the various animals of the farm, and the straw of the cereals
and other waste matter, contains all the elements requisite for plant
life. The litter of fully grown animals, fed upon rich food, with a large
proportion of nitrogenous and phosphatic substances, evidently affords a
more valuable manure than does that of stock fed upon a poorer dietary, or
that of young growing animals. Such manure, too, made under cover, or
sheltered from exposure to rainfall, must also of necessity present a
better quality than when it has been exposed to the open atmosphere and
the washing action of rain. The following table from Stephens shows the
proportions of the ingredients of one ton of farm-yard manure:—
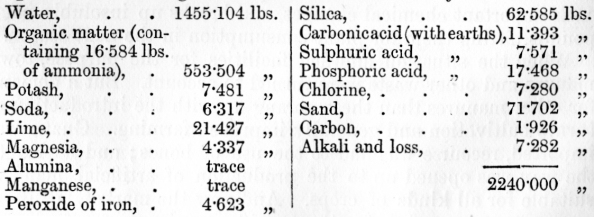
Guano is composed of the excreta of marine fowls and the remains of their
bodies, and it is chiefly found accumulated in thick deposits on several
islands off the Peruvian coast, which are never visited by rain. Their
situation in a rainless zone has prevented the washing away of the
valuable constituents of these strata, which are found consolidated into a
dry unfermented mass. The following is the analysis of a first-class
Peruvian guano:
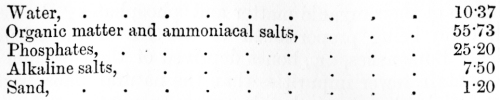
Ammonia contained equal to 18.95.
This shows a most valuable manure, especially
rich both in nitrogenous and phosphatic compounds. Phosphatic bodies
predominate in many guanos, which are thence called phosphatic guanos.
From the excessive demand, the supply of Peruvian guano is rapidly
becoming exhausted. New sources have been made available, but their
geographical position being less favourable, the sample of guano yielded
is much inferior to the Peruvian.
Of the artificial manures, various are
manufactured with a fixed percentage of nitrogenous and phosphatic
compounds. These compounds, however, are for the most part respectively
applied in separate compositions. As nitrogen food, the principal
compounds bestowed upon the soil are the two salts, nitrate of soda and
sulphate of ammonia. Besides these there are many available sources of
nitrogen supply, including all waste organic substances. The phosphoric
acid entering into the composition of artificial manures is entirely
combined with lime, in the form of a salt called phosphate of lime, and
its chief sources are animals' bones, coprolites, and apatite. Of these,
coprolites are found as concretions in certain rock strata, and are
supposed to be the fossilised faeces of gigantic animals, reptiles for the
most part existing at the time of the formation of the strata in question.
Apatite is a mineral phosphate of lime, and is found in large quantity in
various parts of the world. The phosphate of lime as combined in these
quarters consists of two molecules of phosphoric acid in chemical union
with three atoms of calcium, Ca32P04—an almost
insoluble salt. To overcome the insolubility sulphuric acid is added, and
it alters the nature of the phosphate, rendering it soluble, by taking
from it two atoms of calcium—forming with them sulphate of lime or gypsum,
and replacing the calcium atoms by their equivalent of hydrogen. Thus, Ca32PO4
+ 2H2SO4 = 2CaSO4+CaH42P04.
This soluble salt is termed superphoshate of lime. When bones are treated
in this way with sulphuric acid they are known as "dissolved bones;" and
in addition to the phosphate and sulphate of lime, they also possess a
considerable quantity of ammonia, resulting from the organic matter of the
bone. When the gradual absorption of phosphoric acid is desired in the
soil, crushed bones and bone "meal" are applied, without their having
received any such dressing. The minute mechanical division of their
particles permits of a prolonged action upon them by the chemical changes
occurring in the soil, and an equally delayed complete yielding up of
their organic matter and phosphates; the length of such delay being
proportioned to the size of the triturated particles. Bone ashes, i.e.,
bones deprived of their organic substance, contain fewer impurities than
the natural phosphates of lime, and yield the most valuable
superphosphates. "A variety of substances," says Anderson, "are sold under
the name of nitrophosphate, potato manure, cereal manure, &c, which are
all superphosphates, differing only in the proportion of their
ingredients, and in the addition of small quantities of alkaline salts,
sulphate of magnesia, and other substances, but they present little
difference from ordinary superphosphates in their effects." The following,
according to the same authority, are analyses of various superphosphates
:—
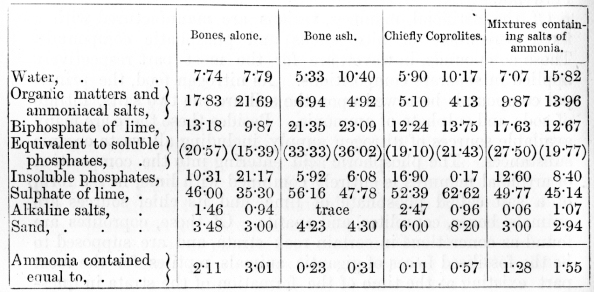
The cheapest source of potash is the mineral
called kainit, containing sulphate of potash, which has now begun to be
extensively supplied to turnips, potatoes, mangolds, &c. Gypsum, or
sulphate of lime, is also applied occasionally to leguminous crops.
Another method for increasing the soil's
fertility employed by advanced farmers, is the feeding of sheep upon rich
artificial substances, whereby, whilst the primary object of fattening the
animals is speedily attained, the soil is benefited by receiving their
valuable excreta, becoming enriched with manurial bodies.
Other bodies, besides, are supplied to the
soil, in order to promote certain physical as well as chemical results.
Thus, clay is added to light sandy and peaty soils, that thereby they may
receive body or staple; and, reversely, sand and peat are added to clay
soils, the former—in order to render them more friable, the latter to
increase their stock of organic matter. In some districts the clay of
strong soils is burnt, for the consequent effect of its friableness being
increased, and many of its insoluble compounds being broken up. Lime,
however, is the principal of all the substances which are added for
combined physical and chemical effects. Besides disintegrating the strong
clay, it also breaks up the insoluble silicates and liberates the combined
alkalies. Moreover, it promotes changes amongst the organic compounds, in
peaty soils especially. Nor has it less importance as being a direct
source of food to plants, for all of them contain more or less lime. By
its application, the quality of grain is improved, and its maturity
hastened; whilst again, in pasture ground, it serves to extirpate moss and
plants of low organisation. All those effects of lime are more marked and
more efficacious if it has been applied in the caustic state. Frequently,
however, it is applied as the carbonate, in the form of chalk, marl, and
shell sand; for these are often to be had cheaply and conveniently, where
lime " shells " are difficult to obtain.
In connection with the above general laws, there are numerous
considerations to be attended to, in regard to the application of manures,
which are too complicated, and too closely associated with actual
practice, to be touched upon here. They bear principally upon the proper
times, quantities, and mutual proportions which are essential to their
profitable use.
Hearing and Management of Stock.—Although, in
our home market, the foreign agriculturists can favourably compete with us
in grain production, it is otherwise with regard to the production of beef
and mutton. It is true, indeed, that, in spite of the obstacles ensured to
them in a ruder system of agricultural practice, and a greater dependence
of necessity on mere natural agencies for the maturing of their stock,
they still can rear cheaper meat. But before our markets are reached,
there are the risks, difficulties, and expenses of transit to be
encountered. Doubtless, the appliances of science will tend to the gradual
diminution of these difficulties, as witness the importation of cooked
beef and mutton of fair quality from Australia, and the sale in some of
our markets at paying figures of fresh beef from America. But before the
American and European countries exporting to us can produce live stock so
speedily attaining a high quality as ours do, and affording such a
high-class description of meat as our markets demand, there must prevail
amongs them a higher practice of agriculture, involving the introduction
of improved breeds of animals. And there is no reason why British farmers
should not maintain their precedence in this respect. Almost the entire
number they export reach us in a lean condition, and require to be
fattened here, under our more advanced system. For since, within recent
times, beef and mutton have become a more important branch of our
agricultural produce than grain, scientific attention has been turned to
the investigation of animal dietary, with the result of many natural
bodies formerly overlooked, together with new artificially prepared
compounds, having come into general use in the feeding of the live stock
of the farm. Physiology has taught us which of the proximate feeding
compounds should be the principal ingredient in the food of each class of
animals. Substances, accordingly, rich in albuminous matter, should
constitute the food of hard-working animals, that fibrin and albumin may
be applied to their muscular wants. To such as it is desired that they
should be quickly fattened, and which undergo only sufficient exercise to
maintain good health, are freely given compounds known to contain much
fat-forming material. To young growing animals a fair proportion of both
kinds of food is offered, and such substances in addition as hold saline
bodies, like phosphate of lime, which avail for the building up of the
bones. Milk affords a safe criterion of the food constituents appropriate
to young animals. It is the food nature provides for them, and none other
can be so perfect. According to Way, the average composition of cow's milk
is—
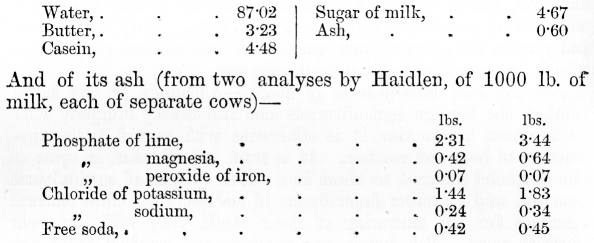
The oleaginous bodies contained in the fluid
are broken up into minute globules or cells; and when new milk is allowed
to settle in any suitable vessel, these rise to the surface, and form the
cream. When the cream is removed, it leaves the skimmed milk almost
entirely devoid of oily matter. The albuminous matter, or casein, is held
in solution by the fluid, by means of some one of the alkaline bodies ;
the latter becomes neutralised by the addition of certain acids, whereupon
the casein coagulates, and this curd, by pressure and suitable treatment,
becomes converted into cheese. The remaining fluid, or whey, contains the
sugar and the inorganic salts. The natural curdling of milk takes place
when lactic acid, C3H6O3, its peculiar
acid, is formed; and its presence is evidenced by the fluid becoming sour
in taste. Johnson says:—"The change which takes place when milk becomes
sour is easily understood. Under the influence of the casein, the elements
of a portion of the milk-sugar are made to assume a new arrangement, and
the sour lactic acid is the results. There is no loss of matter; no new
elements are called into play; nothing is absorbed from the air, or given
off into it; but a simple transposition of the elements of the sugar takes
place, and the new acid compound is produced. These changes appear very
simple, and yet, how difficult is it to conceive by what mysterious
influence the mere contact of this decaying membrane, or of the casein of
the milk, can cause the elements of the sugar to break up their old
connection, and to arrange themselves anew in another prescribed order, so
as to form a compound endowed with properties so very different as those
of lactic acid."
Lactic acid is also produced during the
churning of cream, and is supposed to aid the violent mechanical
agitation, in breaking the walls of the oily cells or globules, after
which the butter separates in mass from the sour butter milk. In the
ordinary domestic manufacture of cheese, " rennet," an acid decoction
prepared from the dried stomach of a calf, is what is ordinarily added to
the milk, for the purpose of coagulating or curdling the casein.
As cow milk is such an invaluable article of
the human dietary, calves are often early deprived of this, their natural
food, or, at all events, only partake of it after its cream has been
abstracted. But suitable artificial food is substituted, rich in the
various proximate constituents. But even in those cases where a fair
amount of milk is afforded to them, it is sometimes usual to mix with it
linseed, or some similar meal, whenever the calf can eat artificial
stuffs, and nutritious green food is provided as well. Lambs and young
pigs are not prematurely deprived of mother's milk; but she is fed with
food calculated to enrich the qualities of her milk.
Before the introduction of turnips, owing to
the want of green or juicy food for the live stock during winter, the
animals had attained, as a rule, their full growth and maturity, ere they
were fattened enough for slaughtering. Throughout summer and the milder
months they had the range of the pasturage; and in winter they were turned
into the fold-yards, more indeed for the purpose of trampling down and
converting the litter into manure, than of being carefully tended, and
provided with nourishing food, their dietary being almost entirely
composed of hay and straw. Sheep also had to subsist entirely upon the
natural pasturage; few, if any, were kept on the arable farms. The state
of matters is now entirely different. A variety of green crops is
cultivated; there is the choice of an immense assortment of artificial
feeding stuffs, and every breed of stock has been improved to the
development of the most extraordinary qualities of speedy and economical
fattening. Consequently, stock is kept in every district in amazingly
increased numbers, and sent to the meat market in prime condition, at a
comparatively early age. Even in such counties, where, from physical and
climatic obstacles, a large proportion of the land necessarily remains in
its natural condition, the cultivation of the residue is wholly directed
towards stock-breeding and rearing, the produce of the large number of
breeding animals kept being sold off as "store" cattle and sheep for
fattening in more favoured districts. In point of fact, since the
increased demand for prime " butcher meat," down through all ranks of the
community, has so particularly raised the price of the article, the great
end of all British agriculture is becoming more and more exclusively the
rearing and feeding of live stock.
The peculiarities of the digestive economy in
ruminants, rendering juicy and bulky food, like our different grasses,
most appropriate to their use, they cannot be reared and fattened on dry
concentrated food alone, without incurring the risk of disease. During
winter and the non-vegetative months our various green and root crops form
admirable substitutes for grasses. Concentrated feeding stuffs added to
the natural bulky food, whilst they do not impair the digestive functions,
supply the absorbents with materials rich in the several proximate
compounds for maintaining and multiplying the animal tissues. As we have
seen by the foregoing analysis, grasses and clovers are much more
nutritious than equal weights of turnips ; wherefore, stock which is being
fattened on grass does not, as a rule, receive concentrated feeding stuff
in addition, though under the high pressure system, the contrary practice
is beginning to obtain. Animals being winter fed, however, receive, as a
matter of course, artificial food in addition to the straw and turnips ;
and their quickly increasing weight is the justification. Stuffs, rich in
albuminous proximate compounds, are the most valuable, by reason of their
affording the necessary flesh-forming material, and at the same time
increasing the manurial value of the excreta; and their value reaches a
maximum by the daily proportionate admixture of oleaginous and respiratory
bodies. Of grains most used are ground barley, beans, and Indian corn;
wheat and oats are not often given to pasturing stock, though the last is
often put before store cattle. Bran, malt dust, and the refuse malt and
liquids of breweries and distilleries, are also made use of. Of the many
waste substances of the manufactory, which chemical knowledge has made
available for stock feeding, the most important are the solid cakes or
tablets, the residuum of the oil-extracting process by pressure from
linseed, rape seed, cotton seed, &c. As these contain some of the oil, and
all the other proximate constituents in the composition of the seed, they
constitute a most invaluable concentrated feeding stuff. It ought not to
be lost sight of, that however high their value, as containing such
constituents may be, it must needs be regulated by their properties of
easy digestion, otherwise such constituents will pass through the
alimentary canal but little acted upon. Digestibility accordingly,
especially in the case of fibrous substances, is greatly promoted by
subjecting feeding stuffs to steaming, or such like concoction, or to
fermentation.
The following table from Anderson, gives the
nutritive composition of numerous feeding materials:—
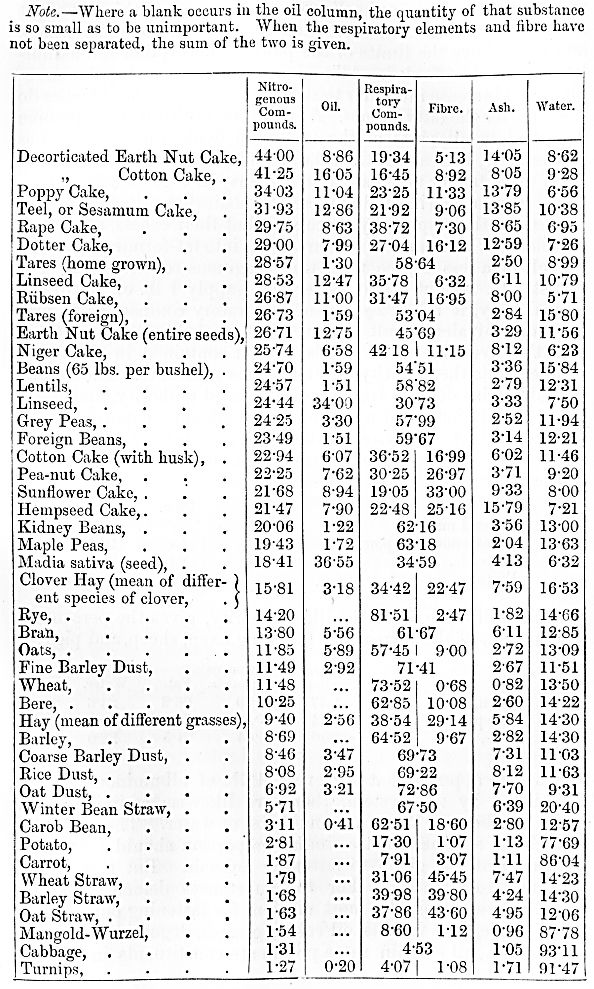
It is obvious that a barely sufficient supply
of food for the daily wear and tear of an animal's existence will not
contribute to any increase of the animal's weight. To produce this last
effect an excess of nutriment over what is requisite for daily wants, and
even above the limits of the power of absorption and assimilation, must be
afforded. Albuminous bodies, as we have seen, replace and increase
muscular tissue, whilst the oleaginous bodies do the same for the fatty
tissues. As the latter multiply, they become deposited in all parts of the
otherwise dense muscular tissues of the body, increasing their bulk and
juiciness, and rendering them more tender and palatable and easy of
digestion as human food. The saccharine and amylaceous bodies are
primarily appropriated for the respiratory processes ; but their excess,
present in the blood, is also capable of conversion into fat-forming
material, though in a less degree than the oleaginous constituents. For
whilst 1 lb. weight of the latter can supply 1lb. of fat to the animal
body, it takes 2½ lbs. of respiratory compounds to produce an equivalent
result. To many it is probably an unsuspected fact, that even in lean
animals, the fat contained in the whole body exceeds the quantity of
albuminous substance. The following table, also derived from the
last-named authority, and based upon calculations resting upon the
elaborate experiments of Messrs Gilbert and Lawes, shows approximately the
general composition of the entire carcass of a lean and a fat animal
respectively:—
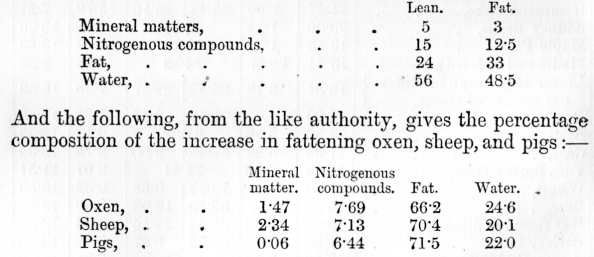
Whence it appears that for every 1lb. of
albuminous matter assimilated by the system, there are likewise
assimilated, in round numbers, 10 lbs. of fat and 3 lbs. of water.
Theoretically, it might be assumed that a similar proportion should
prevail in the food material given to fattening animals. But this is not
sustained in practice. For the substances demonstrated by experience as
being the most efficient for fattening our stock— for example, the various
oil-cakes, grasses, leguminous plants, turnips, &c, all contain these
proximate constituents in a much more equal proportion than that of 1 to
10. Any apparent anomally disappears when we call to mind that with the
progress of —from a physical point of view—degeneration in the animal
body, owing to the want of a natural amount of muscular exertion the
albuminous bodies becomes less and less requisite to the tissues, and
their absorption into the blood slower. This may either be owing to the
already highly nourished and consequently denser condition of the blood
retarding their absorption by the process of endosmoses, or to some
obscure natural provision. At all events, the balance of the blood
constituents is preserved and disease averted by reason thereof. The
increased difficulty of their absorption, however, necessitates a larger
quantity of the albuminous bodies being present in the food, in order that
the absorbents may be enabled to the utmost to take up a sufficient
modicum. All the while the fattening substances, from the ease with which
they are assimilated, have their excess laid up in all parts of the
system. The following table, still from the same authority, shows the
amount of each class of constituents stored in the increase, for every 100
consumed in the food, by—

The last shows the greater power of
assimilation of food possessed by the pig over the sheep, and its
consequent property of cheaper and speedier fattening. A main object in
breed improvement is the development of such a quality in stock.
Farm horses, those admirable serfs of the
husbandman, are appropriately fed with dry concentrated food, rich in
albuminous substances. Experience has selected oats and hay from this
class, with the addition of beans in the spring and early summer months,
when calls for draught power are scarce. In summer the animals enjoy a
short respite, when grass is given, as being-better suited to diminished
muscular expenditure, and economical at the same time.
As regards the actual details of ordinary
agricultural practice and routine, these, indeed, can best be learned by
experience in the field. In every county, nay, almost in every parish,
they vary appreciably. But the scientific principles of agriculture are
equally applicable in every country and clime, and the student may
investigate them to their utmost extent in the works of Stephen's;
Wilson's Farm Crops; Liebig's, Anderson's, and Johnston's writings on
Chemistry applied to Agriculture; Roscoe on Chemistry; Geikie on Geology;
and Balfour and Brown on Botany, with many other volumes too numerous to
mention. |